International Symposium on Correlated Electrons | October 5th - 7th, 2021
On October 5th -7th, 2021, the 2021 International Symposium on Correlated Electrons (SymCorrel21) will take place online. This international and interdisciplinary symposium brings together experts from the different subfields of the quantum sciences to elaborate on systematic theoretical and computational approaches to interacting many-electron quantum systems in quantum many-body physics, particularly quantum chemistry. The more concise quantum information theoretical perspective on quantum correlations will play a central role in that context.
Confirmed Speakers
Ignacio Cirac - Max Planck Institute for Quantum Optics
Fermionic lattice Systems with Permutation Symmetry
We study the ground states of lattice Hamiltonians that are invariant under permutations, in the limit where the number of lattice sites diverges. For spin systems, these are product states, a fact that follows directly from the quantum de Finetti theorem. For fermionic systems, however, the problem is very different, since mode operators acting on different sites do not commute, but anti-commute. We construct a family of fermionic states, from which such ground states can be easily computed. They are characterized by few parameters whose number only depends on M, the number of modes per lattice site. We also derive the phase diagram of the Hubbard Harmiltonian with permutation symmetry. Work in collaboration with: Jiří Guth Jarkovský and Lorenzo Piroli.
Laura Gagliardi - University of Chicago
Active-Space Based Methods for Strongly Correlated Systems
I will give an overview of the challenges that modern electronic structure theory faces in de- scribing strongly correlated chemical systems from molecules to materials. I will describe our efforts in developing active-space based techniques for extended systems. We recently proposed a method to obtain a new kind of multiconfiguration wave function called localized active space self-consistent field, LASSCF. With LASSCF, the entire active space is decomposed in multiple unentangled active subspaces that are localized on user-defined fragments [1]. I will also present our recent development of density matrix embedding methods based on multireference solvers for molecules and materials [2], [3].
[1] M. R. Hermes, R. Pandharkar, and L. Gagliardi, The Variational Localized Active Space Self-Consistent Field Method, J. Chem. Theory Comput., 16, 2020, 4923-4937
[2] H. Q. Pham, M. R. Hermes, L. Gagliardi, Periodic Electronic Structure Calculations with Density Matrix Embedding Theory J. Chem. Theory Comput., 16, 2020, 130-140
[3] A. Mitra, H. Pham R. Pandharkar, M. Hermes, L. Gagliardi, Excited States of Crystalline Point Defects with Multireference Density Matrix Embedding Theory, Submitted
Eugene DePrince - Florida State University
Reduced-density-matrix-based descriptions of dynamic and nondynamic electron correlation
The direct variational optimization of the two-electron reduced density matrix (2RDM) can provide a reference-independent description of the electronic structure of many-electron systems that naturally captures strong or nondynamic electron correlation effects. I will discuss the variational 2RDM (v2RDM) approach as an approximate solver for the complete active space self consistent field (CASSCF) and doubly occupied configuration interaction (DOCI) methods, as well as post-v2RDM strategies to capture dynamic correlation effects lacking in CASSCF and DOCI.
Lexin Ding - LMU Munich
Orbital Entanglement and Correlation
A recent development in quantum chemistry has established the quantum mutual information between orbitals as a major descriptor of electronic structure. This has already facilitated remarkable improvements of numerical methods and may lead to a more comprehensive foundation for chemical bonding theory. In this introductory talk, I will review key concepts from quantum information theory, and discuss the challenge in applying these concepts to systems of electrons. In particular, we are able to separate the total correlation between two orbitals into classical and quantum part, the latter of which contains the important entanglement. We apply this versatile toolbox to ground states of molecules with different reference bases, including the Hartree-Fock orbitals and the newly introduced, fully localised, atomic-like molecular orbitals. Our analysis reveals a deep connection between chemical bonding and orbital entanglement, and the effect of orbital localisation on the correlation pattern in the molecular ground state.
Hardy Gross - University of Jerusalem
DFT, RDMFT, and the exact electron factorization
Three approaches to electron correlation will be highlighted in this lecture: Density Functional Theory (DFT), Reduced Density Matrix Functional Theory (RDMFT) and the exact factoriza- tion. After an overview of the basic concepts of ground-state DFT as well as time-dependent DFT, the description of quantum phases, such as magnetism and superconductivity will be addressed within this framework. The idea is to include the order parameter describing the re- spective phases, in addition to the density, explicitly in the energy functional of DFT. Systems with strong correlations, such as Mott insulators and stretched H2, cannot be described cor- rectly within DFT, at least not with standard functionals. To tackle strongly correlated systems, Reduced-Density-Matrix-Functional Theory (RDMFT) and the exact electron factorization will be presented as viable alternatives to DFT. The latter allows one to split a many-electron system into subsystems (such as strongly correlated and weakly correlated electrons) in a formally exact fashion. This ultimately leads to novel embedding algorithms which, as opposed to most other embedding strategies, permit the treatment of long-range correlations.
Sabre Kais - Purdue University
Quantum Machine-Learning for Electronic Structure Calculations
Quantum machine learning algorithms have emerged to be a promising alternative to their classical counterparts as they leverage the power of quantum computers. In this talk, I will present our developed quantum algorithm that can be used to obtain accurate ground and excited states for molecules and two-dimensional materials. Our technique is based on a shallow neural network encoding the desired state of the system with the amplitude computed by sampling the Gibbs- Boltzmann distribution using a quantum circuit with the resource requirements of our algorithm is strictly quadratic. Then, I will show our implementation of the developed algorithm on the actual IBM-Q quantum devices. The results of the quantum simulations for simple molecules and two-dimensional materials such as graphene and transition metal-dichalcogenides are in good agreement with the results procured from conventional electronic structure calculations. The quantum machine learning approach is general and can be used to calculate band structure different materials. (1) “Quantum Machine Learning for Electronic Structure Calculations” Xia, Rongxin; Kais, Sabre, Nature Comm. 9, 4195 (2018) (2) “Quantum Machine-Learning for Eigenstate Filtration in Two-Dimensional Materials”, Sureshbabu, Shree Hari; Sajjan, Sangchul; Kais, Sabre, arXiv:2105.09488 (2021) (3) “Implementation of Quantum Machine Learning for Electronic Structure Calculations of Periodic Systems on Quantum Computing Devices”, Sureshbabu, Shree Hari; Sajjan, Manas; Oh, Sangchul; Kais, Sabre, J. Chem. Inf. 61, 2667-2674 (2021)
Stefan Knecht - GSI Darmstadt
Shedding Light on the Chemistry of (Super-)heavy Elements with Electronic Structure Theory
Computational modeling is an integral part of chemical research. For instance, providing a detailed understanding of the nature of a chemical bond and how it relates to the chemical reactivity of a molecule is of central interest in chemistry. To rationalize such properties at a molecular level requires a tailor-made quantum chemical framework that provides the means to accurately describe the central piece of information relevant to chemistry, viz., the electronic structure. Its determination relies on the solution of the quantum mechanical equations for the electronic many-body problem. Whereas solving the electronic Schr ̈odinger equation (often) yields sufficiently accurate results for light elements, for example those of the upper part of the periodic table, this is generally not the case for (super-)heavy elements. For the latter, an explicit consideration of the theory of special relativity is required. In this contribution, the develop- ment and application of time-independent multiconfigurational wave-function based quantum chemical methods [1-7] as well as real-time time-dependent multiconfigurational approaches [8] to the calculation of electronic and magnetic properties of molecular complexes in their ground- and electronically excited states is presented that are designed to accommodate the needs to account for the correlated motion of the elec- trons as well as for relativistic effects in a rigorous manner. Moreover, I will discuss our recent development of a genuine two-component Hamilto- nian framework which enables for the first time a simultaneously highly accurate description of core as well as valence molecular properties of (super-)heavy elements.
̈
[1] S.Knecht, O. Legeza, and M. Reiher, J.Chem.Phys.,140, 041101(2014).
[2] S. Knecht, S. Keller, J. Autschbach, M. Reiher, J. Chem. Theory Comput., 12, 5881 (2016). [3] S. Battaglia, S. Keller, and S. Knecht, J. Chem. Theory Comput., 14, 2353 (2018).
[4] S. Knecht, H. J. Aa. Jensen and T. Saue, Nat. Chem.,11, 40 (2019).
[5] L. Freitag, A. Baiardi, S. Knecht, and L. Gonzalez, submitted, 2021, chemrxiv-2021-brv78. [6] S. Knecht, in preparation, 2021.
[7] C. Hoyer, H. Hu, S. Knecht and X. Li, in preparation, 2021.
[8] A. Baiardi and S. Knecht, in preparation, 2021.
[9] S. Knecht, M. Repisky, H. J. Aa. Jensen and T. Saue, in preparation, 2021.
Peter Knowles - Cardiff University
Multiconfigurational methods for excited states
In this talk, I will first introduce and review the multiconfiguration approach to computational molecular electronic structure. I will then discuss some recent developments in effectiveness and efficiency in solving the multi configurational SCF problem, and discuss some examples. Finally, I will present a scheme to include vibronic and polaritonic effects directly by extending the chosen electron-only many-body method.
Örs Legeza - Wigner Research Center Budapest
Tensor network state methods in
material science and ab initio quantum chemistry
In this contribution, we overview tensor network states techniques that can be used for the treatment of high-dimensional optimization tasks used in many-body quantum physics with long range interactions and ab initio quantum chemistry. We will discuss the controlled manipulation of entanglement in light of fermionic orbital optimization. Recent developments on tree-tensor network states, multipartite entanglement, time-dependent variational principle (TDVP), exter- nally corrected coupled cluster density matrix renormalization group (DMRG-TCCSD) method, will be discussed in more detail. Finally, some new results will be shown for extended periodic systems in one- and two dimensions, for topologically protected end spins in carbon nanotubes, for transition metal complexes, graphene nanoribbons and Wigner crystals.
Christian Ochsenfeld - LMU Munich
Linear-scaling methods for solving the Schrödinger equation of complex systems
Solving the Schrödinger equation is key to the quantum-mechanical description of molecular mat- ter in an exact non-relativistic fashion. While an analytic solution is for many-electron systems impossible, a hierarchy of approximations has been introduced over many decades to system- atically converge towards the exact solution in accounting for the decisive electron-correlation effects. This hierarchy of approximations allows in principle to systematically check the reli- ability of computational predictions, so that quantum-chemical calculations have become an important tool for gaining deeper insights into molecular systems. However, the applicability to large systems is hampered by the strong polynomial increase of the computational effort with system size. Therefore the central goal of our work is to overcome this scaling wall and to develop linear- and for specific properties even sublinear-scaling methods. In combination with low prefactor implementations on both CPUs and GPUs, this opens up new possibilities for computing large and complex systems.
Prineha Narang - Harvard University
Quantum Information and Algorithms for Correlated Quantum Matter
Piotr Piecuch - Michigan University
Approaching Exact Quantum Chemistry by Semi-Stochastic and Selected-CI-Driven Coupled-Cluster Computations
It is well established that the exponential wave function ansatz of coupled-cluster (CC) theory and its extensions to excited, open-shell, and multireference states are among the most efficient ways of incorporating many-electron correlation effects in molecular applications. In this talk, after a brief overview of the single- and multireference CC approaches, I will discuss novel ways of obtaining accurate energetics equivalent to high-level CC calculations, such as CCSDT, CCSDTQ, and EOMCCSDT, at small fractions of the computational costs, even when multireference correlation effects become significant, which result from the merger of moment expansions defining the CC(P;Q) formalism [1] with Quantum Monte Carlo (QMC) propaga- tions in the many-electron Hilbert space [2,3] and with the selected configuration interaction (CI) approach abbreviated as CIPSI [4]. Time permitting, I will also demonstrate that one can use the merger of the CC and stochastic and selected CI ideas to extract the exact, full CI (FCI), or near-exact energetics out of the early stages of FCIQMC propagations or from the initial iterations of CIPSI with the help of the relatively inexpensive polynomial steps similar to CCSD, eliminating exponential complexity of conventional FCI Hamiltonian diagonalizations altogether [5,6].
[1] J. Shen and P. Piecuch, Chem. Phys. 401, 180 (2012); J. Chem. Phys. 136, 144104 (2012); N. P. Bauman, J. Shen, and P. Piecuch, Mol. Phys. 115, 2860 (2017).
[2] J. E. Deustua, J. Shen, and P. Piecuch, Phys. Rev. Lett. 119, 223003 (2017); J. Chem. Phys. 154, 124103 (2021).
[3] J. E. Deustua, S. H. Yuwono, J. Shen, and P. Piecuch, J. Chem. Phys. 150, 111101 (2019); S. H. Yuwono, A. Chakraborty, J. E. Deustua, J. Shen, and P. Piecuch, Mol. Phys. 118, e1817592 (2020).
[4] K. Gururangan, J. E. Deustua, J. Shen, and P. Piecuch, J. Chem. Phys., submitted; arXiv:2107.10994.
[5] J. E. Deustua, I. Magoulas, J. Shen, and P. Piecuch, J. Chem. Phys. 149, 151101 (2018); J. J. Eriksen et al., J. Phys. Chem. Lett. 11, 8922 (2020).
[6] I. Magoulas, K. Gururangan, P. Piecuch, J. E. Deustua, and J. Shen, J. Chem. Theory Comput. 17, 4006 (2021).
Mario Piris - Ikerbasque
From 1RDMFT to NOF Approximations
Appropriate representations of the electronic structure of atoms, molecules and solids without explicit recourse to the N-particle density matrix can alternatively be obtained by the one- particle reduced density matrix (1RDM) functional theory [1], where the 1RDM is used directly for ground-state variational calculations. The functional E[1RDM] is defined independently of the external potential and is therefore a universal functional. In the first part of this presentation, an overview of the genuine 1RDMFT will be given. Computational schemes based on the exact constrained search formulation are too expensive; so the 1RDM functional requires a practical approach. For a Hamiltonian involving no more than two-body interactions, it is well known that the ground-state energy can be cast as an exact functional of the two-particle reduced density matrix (2RDM). We actually only need to reconstruct the electron-electron potential energy Vee in terms of the 1RDM, since the non-interacting part of the electronic Hamiltonian is a one-particle operator. Unfortunately, the explicit reconstruction of Vee in terms of the 1RDM has resulted in an unattainable goal so far, and we have to settle for making approximations. In practical applications, we employ the exact energy functional but using an approximate 2RDM that is built from the 1RDM. Approximating the energy functional has important consequences: the theorems obtained for the exact functional of the 1RDM are no longer valid. As a consequence, the functional N-representability problem arises [2]. The functionals currently in use are constructed in the basis where the 1RDM is diagonal, which is the definition of a natural orbital functional (NOF). So far, several approximate functionals have been proposed [3-5] and an open source NOF-based software is available [6] for quantum chemistry calculations. In the second part of the talk, the state-of-the-art in the approximate NOF theory will be presented.
[1] T. L. Gilbert, Phys. Rev. B 12, 2111 (1975); M. Levy, Proc. Natl. Acad. Sci. USA 76, 6062 (1979); S. M. Valone, J. Chem. Phys. 73, 1344 (1980).
[2] M. Piris, in Many-body approaches at dierent scales: a tribute to N. H. March on the occasion of his 90th birthday, Chap. 22, pp. 231-247. New York: Springer (2018).
[3] M. Piris, Adv. Chem. Phys. 134, 387-427 (2007).
[4] K. Pernal and K. J. H. Giesbertz, Top Curr Chem 368, 125-184 (2016).
[5] I. Mitxelena, M. Piris, J. M. Ugalde, Adv. Quantum Chem. 79, 155-177 (2019). [6] M. Piris, I. Mitxelena, Comp. Phys. Comm. 259, 107651 (2021).
Uli Schollwöck - LMU Munich
Introduction to MPS
This talk is to set the stage for people without or little background in the framework of matrix product states (MPS) to show how they work as tools for the simulation for quantum many-body systems in low dimensions.
Gustavo Scuseria - Rice University
Geminal Theories for Strong Correlation
The antisymmetrized geminal power (AGP) is not a great wavefunction for chemistry. Electrons like to form pairs but geminals are hardly all the same as in AGP, the number projected BCS wavefunction. We also know that in quantum chemistry, spin projection is much more important than projecting number of electrons, a symmetry that does not break spontaneously in mean-field. So why bother with AGP? We have recently shown that AGP and its seniority zero (paired) excitations are a great starting point for describing fancy geminal wave functions with polynomial computational cost. In this presentation, I will discuss our current progress with this model. A few recent papers [1-6] describe some of the formal aspects of the theory. The extension to broken pairs is currently under development. [1] Geminal-based configuration interaction, T. M. Henderson, and G. E. Scuseria, J. Chem. Phys. 151, 051101 (2019); http://arxiv.org/abs/1906.11361 [2] Efficient evaluation of AGP reduced density matrices, A. Khamoshi, T. M. Henderson, and G. E. Scuseria, J. Chem. Phys. 151, 184103 (2019); https://arxiv.org/abs/1909.06345 [3] Correlating the Antisymmetrized Geminal Power Wave Function, T. M Henderson and G. E. Scuseria, J. Chem. Phys. 153, 084111 (2020); https://arxiv.org/abs/2007.03671 [4] Geminal replacement models based on AGP, R. Dutta, T. M Henderson, and G. E. Scuseria, J. Chem. Theory Comput. 16, 6358 (2020); https://arxiv.org/abs/2008.00552 [5] Exploring non-linear correlators on AGP, A. Khamoshi, G. Chen, T. M. Henderson, and G. E. Scuseria, J. Chem. Phys. 154, 074113 (2021); https://arxiv.org/abs/2012.02377 [6] Construction of linearly independent non-orthogonal AGP states, R. Dutta, G. Chen, T. M. Henderson, and G. E. Scuseria, J. Chem. Phys. 154, 114112 (2021); https://arxiv.org/abs/2101.08911
Frank Verstraete - University of Gent
Constructing convex sets of local expectation values in correlated many-body systems
Weitao Yang - Duke University
Describing Strong Correlation in Density Functional Theory
Progress to describing strong correlation within density functional theory will first be summarized. Then the focus will be on the two approaches: The fractional spin localized orbital scaling correction (FSLOSC) and the multireference DFT based on linear response theory.
In FSLOSC, we add to commonly used density functional approximation (DFA) with a frac- tional spin correction developed from recently developed localized orbital scaling correction (LOSC). FSLOSC introduces the explicit derivative discontinuity and largely restores the flat- plane conditions of electronic energy at fractional charges and fractional spins, which are nec- essary conditions for functional approximation to describe strong correlation. In addition to improving results from conventional functionals for the prediction of ionization potentials, elec- tron affinities, quasiparticle spectra, and reaction barrier heights, FSLOSC properly describes the dissociation of ionic species, single bonds, and multiple bonds without breaking space or spin symmetry and corrects the spurious fractional-charge dissociation of heteroatom molecules of conventional functionals. FSLOSC demonstrates success in reducing delocalization error and including strong correlation, within low-cost density functional approximation.
In the multireference DFT, we developed an alternative approach within DFT, which uses a generalized auxiliary system that is of a different symmetry, such as particle number or spin, from that of the physical system. The total energy of the physical system consists of two parts: the energy of the auxiliary system, which is determined with a chosen density functional ap- proximation (DFA), and the excitation energy from an approximate linear response theory that restores the symmetry to that of the physical system, thus rigorously leading to a multidetermi- nant description of the physical system. The electron density of the physical system is different from that of the auxiliary system and is uniquely determined from the functional derivative of the total energy with respect to the external potential. Our energy functional is thus an im- plicit functional of the physical system density, but an explicit functional of the auxiliary system density. We show that the total energy minimum and stationary states, describing the ground and excited states of the physical system, can be obtained by a self-consistent optimization with respect to the explicit variable, the generalized Kohn-Sham noninteracting density matrix. We have developed the generalized optimized effective potential method for the self-consistent optimization. Among options of the auxiliary system and the associated linear response the- ory, reformulated versions of the particle-particle random phase approximation (pp-RPA) and the spin-flip time-dependent density functional theory (SFTDDFT) are selected for illustration of principle. Numerical results show that our multireference DFT successfully describes static correlation in bond dissociation and double bond rotation.
(1) Su, N. Q.; Li, C.; Yang, W. Describing Strong Correlation with Fractional-Spin Cor- rection in Density Functional Theory. Proc Natl Acad Sci USA 2018, 115 (39), 9678–9683. https://doi.org/10/gd8gr8.
(2) Chen, Z.; Zhang, D.; Jin, Y.; Yang, Y.; Su, N. Q.; Yang, W. Multireference Density Func- tional Theory with Generalized Auxiliary Systems for Ground and Excited States. The Journal of Physical Chemistry Letters 2017, 4479–4485. https://doi.org/10.1021/acs.jpclett.7b01864.
Dominika Zgid - University of Michigan
I will discuss newest developments in the Green's function methods for solids. Strongly correlated solids display many characteristic phases and phase transitions. For di?fferent phases present in solids di?erent groups of orbitals are di?fferently occupied and the orbital energy ordering changes signifi?cantly. In this talk, I will discuss di?fferent strongly correlated solids such as SrMnO3,
NdNiO2, and BiVO3. I will focus on di?fferences in the band structure, orbital occupation, and the orbitals near the Fermi level obtained within self-consistent GW and the self-energy embedding theory (SEET) as a function of temperature.
Your talk at SymCorrel21
Besides the many excellent invited talks, we invite all participants to submit a title and abstract for a recorded contributed talk. The accepted contributed talks will be available, on-demand, to all participants on the event platform. Depending on the availability of slots, some talks might be upgraded to contributed live talks. Various contributed speakers will be given the opportunity to introduce themselves and to advertise their talk during the flash session on the first day.
Talk submissions can be done via the registration form. Submission deadline is September 24th.
Local Organization
- Lexin Ding
- Julia Liebert
- Jan Niklas-Boyn
- LeeAnn Sager
Venue
The workshop will be held online, using the platform "MeetAnyway" of the MCQST.
Contact
symcorrel21[at]mcqst.de
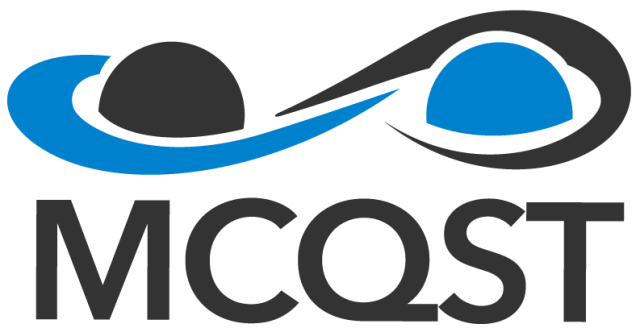
Thank you
The organizers thank the Munich Center for Quantum Science and Technology (MCQST) for the support in hosting the symposium.