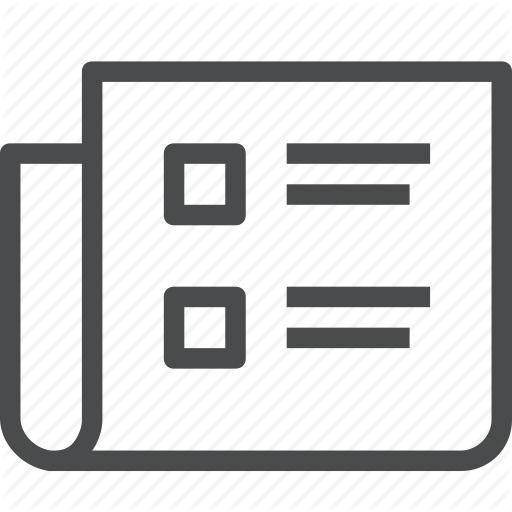
Simulating Optical Single Event Transients on Silicon Photonic Waveguides for Satellite Communication
G. Terrasanta, M. W. Ziarko, N. Bergamasco, M. Poot, J. Poliak
Ieee Transactions on Nuclear Science 71 (2), 176-183 (2024).
Photonic integrated circuits (PICs) are a promising platform for space applications. In particular, they have the potential to reduce the cost, size, weight, and power (C-SWaP) consumption of satellite payloads that employ free-space optical communication. However, the effect of the space environment on such circuits has yet to be fully understood. Here, a simulation framework to investigate the impact of heavy ions on a silicon photonic waveguide is presented. These high-energy particles temporarily increase the waveguide losses, resulting in a drop of the transmitted power, commonly defined as either optical single event transient (OSET) or single event effect (SEE). The magnitude and rate of such transients are simulated. The framework is based on three open-source tools: OMERE, Geant4, and Meep. First, the heavy ion fluxes are modeled for commonly used satellite orbits. Afterward, Monte Carlo simulations are used to generate realistic ion tracks and their effect is evaluated with 3-D finite-difference time-domain simulations. The results show that SEEs have only a small impact on the transmission properties of silicon waveguides in the simulated orbits, thus indicating the potential of using silicon PICs in the space environment. Furthermore, the importance of having realistic carrier distributions, compared to using only an analytical model, is discussed.
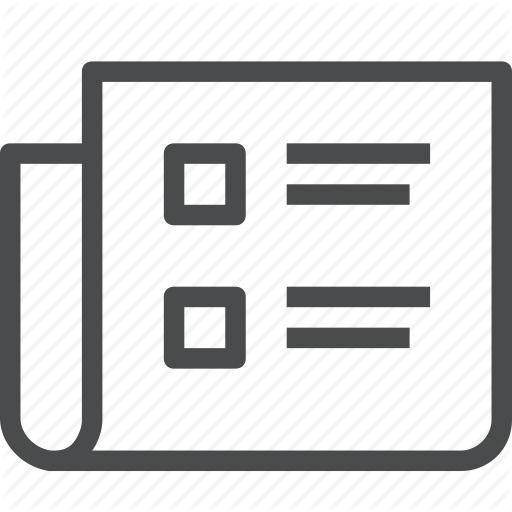
Efficient adiabatic-coupler-based silicon nitride waveguide crossings for photonic quantum computing
T. Sommer, N. Mange, P. Wegmann, M. Poot
Optics Letters 48 (11), 2981-2984 (2023).
Optical integrated quantum computing protocols, in partic-ular using the dual-rail encoding, require that waveguides cross each other to realize, e.g., SWAP or Toffoli gate oper-ations. We demonstrate efficient adiabatic crossings. The working principle is explained using simulations, and sev-eral test circuits are fabricated in silicon nitride (SiN) to characterize the coupling performance and insertion loss. Well-working crossings are found by experimentally varying the coupler parameters. The adiabatic waveguide crossing (WgX) outperforms a normal directional coupler in terms of spectral working range and fabrication variance stability. The insertion loss is determined using two different meth-ods: using the transmission and by incorporating crossings in microring resonators. We show that the latter method is very efficient for low-loss photonic components. The lowest insertion loss is 0.18 dB (4.06%) enabling high-fidelity NOT operations. The presented WgX represents a high-fidelity (96.2%) quantum NOT operation. © 2023 Optica Publishing Group
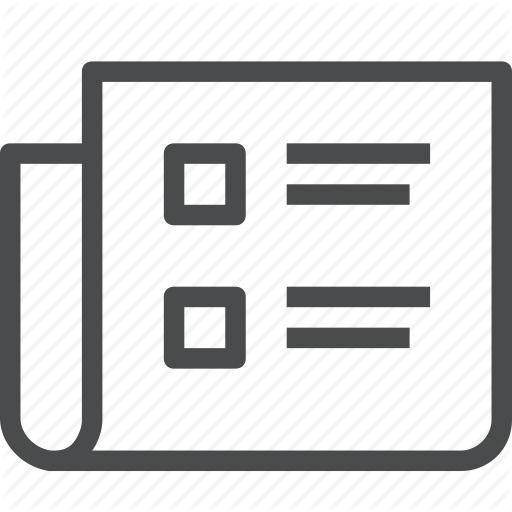
Relaxation and dynamics of stressed predisplaced string resonators
X. Yao, D. Hoch, M. Poot
Physical Review B 106 (17), 174109 (2022).
Predisplaced micromechanical resonators made from stressed materials give rise to new static and dynamic behavior, such as geometric tuning of stress. Here, an analytical model is presented to describe the mechanics of such predisplaced resonators. The bending and tension energies are derived and a modified Euler-Bernoulli equation is obtained by applying the least action principle. By projecting the model onto a cosine shape, the energy landscape is visualized, and the predisplacement dependence of stress and frequencies is studied semianalytically. The analysis is extended with finite-element simulations, including the mode shapes, the role of overhang, the stress distribution, and the impact of film stress on beam relaxation.
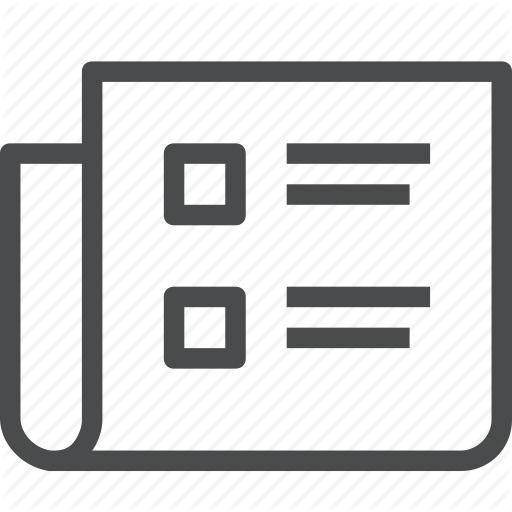
Geometric Tuning of Stress in Predisplaced Silicon Nitride Resonators
D. Hoch, X. Yao, M. Poot
Nano Letters 22 (10), 4013-4019 (2022).
We introduce a novel method to geometrically tune the tension in prestrained resonators by making Si3N4 strings with a designed predisplacement. This enables us, for example, to study their dissipation mechanisms, which are strongly dependent on the stress. After release of the resonators from the substrate, their static displacement is extracted using scanning electron microscopy. The results match finite-element simulations, which allows a quantitative determination of the resulting stress. The in- and out-of-plane eigenmodes are sensed using on-chip Mach-Zehnder interferometers, and the resonance frequencies and quality factors are extracted. The geometrically controlled stress enables tuning not only of the frequencies but also of the damping rate. We develop a model that quantitatively captures the stress dependence of the dissipation in the same SiN film. We show that the predisplacement shape provides additional flexibility, including control over the frequency ratio and the quality factor for a targeted frequency.
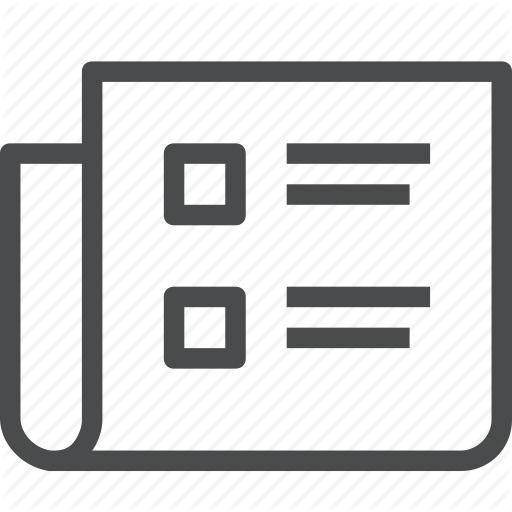
Aluminum nitride integration on silicon nitride photonic circuits: a hybrid approach towards on-chip nonlinear optics
G. Terrasanta, T. Sommer, M. Muller, M. Althammer, R. Gross, M. Poot
Optics Express 30 (6), 8537-8549 (2022).
Aluminum nitride (AlN) is an emerging material for integrated quantum photonics due to its large chi((2)) nonlinearity. Here we demonstrate the hybrid integration of AlN on silicon nitride (SiN) photonic chips. Composite microrings are fabricated by reactive DC sputtering of caxis oriented AlN on top of pre-patterned SiN. This new approach does not require any patterning of AlN and depends only on reliable SiN nanofabrication. This simplifies the nanofabrication process drastically. Optical characteristics, such as the quality factor, propagation losses and group index, are obtained. Our hybrid resonators can have a one order of magnitude increase in quality factor after the AlN integration, with propagation losses down to 0.7 dB/cm. Using finite-clement simulations, phase matching in these waveguides is explored. (C) 2022 Optica Publishing Group under the terms of the Optica Open Access Publishing Agreement
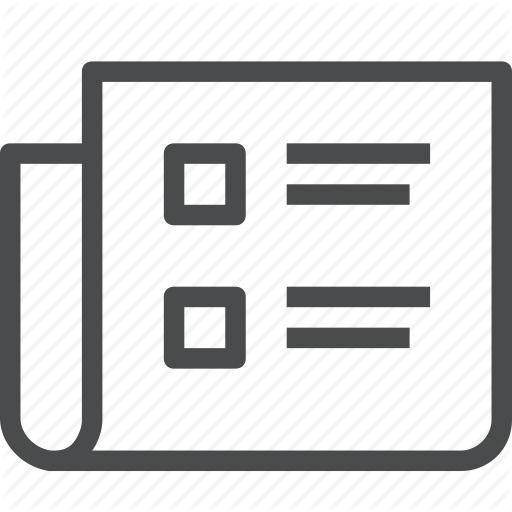
Control over Light Emission in Low-Refractive-Index Artificial Materials Inspired by Reciprocal Design
L. Maiwald, T. Sommer, M. S. Sidorenko, R. R. Yafyasov, M. E. Mustafa, M. Schulz, M. V. Rybin, M. Eich, A. Y. Petrov
Advanced Optical Materials 10 (1), 2100785 (2022).
Reciprocal space engineering allows tailoring the scattering response of media with a low refractive-index contrast. Here it is shown that a quasiperiodic leveled-wave structure with well-defined reciprocal space and random real space distribution can be engineered to open a complete photonic bandgap (CPBG) for any refractive-index contrast. For these structures, an analytical estimation is derived, which predicts that there is an optimal number of Bragg peaks for any refractive-index contrast. A finite 2D or 3D CPBG is expected at this optimal number even for an arbitrarily small refractive-index contrast. Results of numerical simulations of dipole emission in 2D and 3D structures support the estimations. In 3D simulations, an emission suppression of almost 10 dB is demonstrated with a refractive index down to 1.38. The 3D structures are realized by additive manufacturing on millimeter scale for a material with a refractive index of n approximate to 1.59. Measurements confirm a strong suppression of microwave transmission in the expected frequency range.
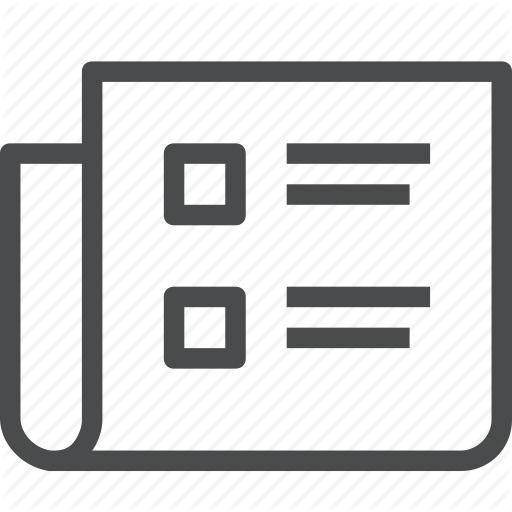
Efficient Optomechanical Mode-Shape Mapping of Micromechanical Devices
D. Hoch, K. J. Haas, L. Moller, T. Sommer, P. Soubelet, J. J. Finley, M. Poot
Micromachines 12 (8), 880 (2021).
Visualizing eigenmodes is crucial in understanding the behavior of state-of-the-art micromechanical devices. We demonstrate a method to optically map multiple modes of mechanical structures simultaneously. The fast and robust method, based on a modified phase-lock loop, is demonstrated on a silicon nitride membrane and shown to outperform three alternative approaches. Line traces and two-dimensional maps of different modes are acquired. The high quality data enables us to determine the weights of individual contributions in superpositions of degenerate modes.
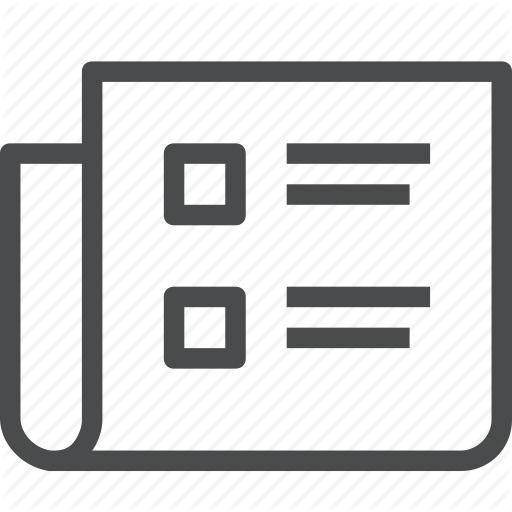
Growth of aluminum nitride on a silicon nitride substrate for hybrid photonic circuits
G. Terrasanta, M. Müller, T. Sommer, S. Geprägs, R. Gross, M. Althammer, M. Poot
Materials for Quantum Technology 1, 21002 (2021).
Aluminum nitride (AlN) is an emerging material for integrated quantum photonics with its excellent linear and nonlinear optical properties. In particular, its second-order nonlinear susceptibility χ(2) allows single-photon generation. We have grown AlN thin films on silicon nitride (Si3N4) via reactive DC magnetron sputtering. The thin films have been characterized using x-ray diffraction (XRD), optical reflectometry, atomic force microscopy (AFM), and scanning electron microscopy. The crystalline properties of the thin films have been improved by optimizing the nitrogen to argon ratio and the magnetron DC power of the deposition process. XRD measurements confirm the fabrication of high-quality c-axis oriented AlN films with a full width at half maximum of the rocking curves of 3.9° for 300 nm-thick films. AFM measurements reveal a root mean square surface roughness below 1 nm. The AlN deposition on SiN allows us to fabricate hybrid photonic circuits with a new approach that avoids the challenging patterning of AlN.
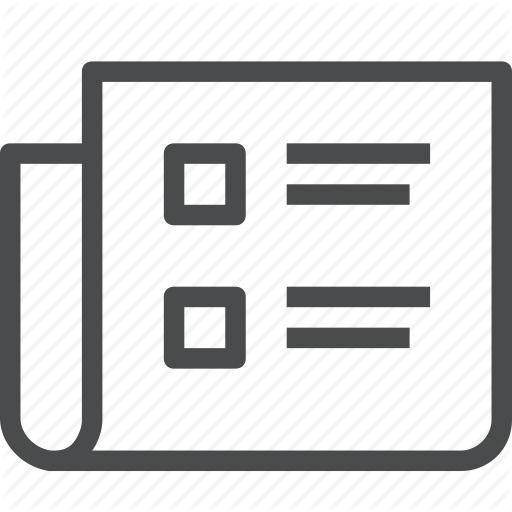
Hybrid Aluminum Nitride Integration on Silicon Nitride Photonic Circuits
G. Terrasanta, M. Muller, T. Sommer, M. Althammer, M. Poot, Ieee
2021 Conference on Lasers and Electro-Optics (CLEO), San Jose, CA, USA (2021).
We demonstrate sputtering of high-quality aluminum nitride films onto prefabricated silicon nitride photonic circuits, simplifying their nanofabrication. Hybrid microring devices show reduced bending loss and low propagation losses, enabling future on-chip quantum optics experiments.
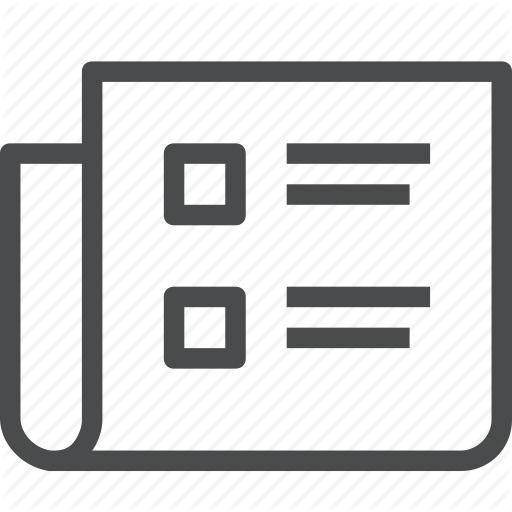
On-chip quantum optics and integrated optomechanics
D. Hoch, T. Sommer, S. Muller, M. Poot
Turkish Journal of Physics 44 (3), 239-246 (2020).
Recent developments in quantum computing and the growing interest in optomechanics and quantum optics need platforms that enable rapid prototyping and scalability. This can be fulfilled by on-chip integration, as we present here. The different nanofabrication steps are explained, and our automated measurement setup is discussed. We present an opto-electromechanical device, the H-resonator, which enables optomechanical experiments such as electrostatic springs and nonlinearities and thermomechanical squeezing. Moreover, it also functions as an optomechanical phase shifter, an essential element for our integrated quantum optics efforts. Besides this, the equivalent of a beam splitter in photonics-the directional coupler-is shown. Its coupling ratio can be reliably controlled, as we show with experimental data. Several directional couplers combined can realize the CNOT operation with almost ideal fidelity.