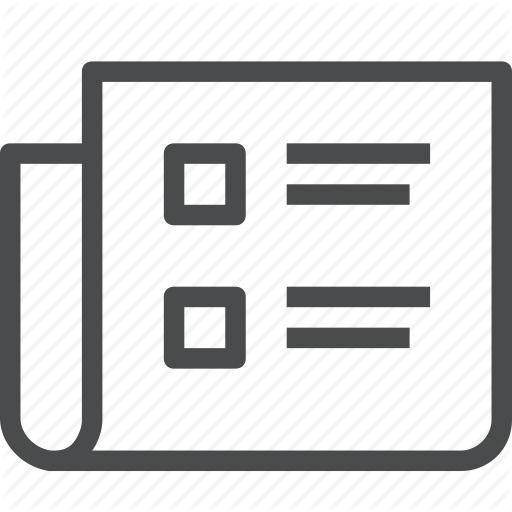
Realizing Altermagnetism in Fermi-Hubbard Models with Ultracold Atoms
P. Das, V. Leeb, J. Knolle, M. Knap
Physical Review Letters 132 (26), 263402 (2024).
Altermagnetism represents a type of collinear magnetism, that is in some aspects distinct from ferromagnetism and from conventional antiferromagnetism. In contrast to the latter, sublattices of opposite spin are related by spatial rotations and not only by translations and inversions. As a result, altermagnets have spin-split bands leading to unique experimental signatures. Here, we show theoretically how a d-wave altermagnetic phase can be realized with ultracold fermionic atoms in optical lattices. We propose an altermagnetic Hubbard model with anisotropic next-nearest neighbor hopping and obtain the Hartree-Fock phase diagram. The altermagnetic phase separates in a metallic and an insulating phase and is robust over a large parameter regime. We show that one of the defining characteristics of altermagnetism, the anisotropic spin transport, can be probed with trap-expansion experiments.
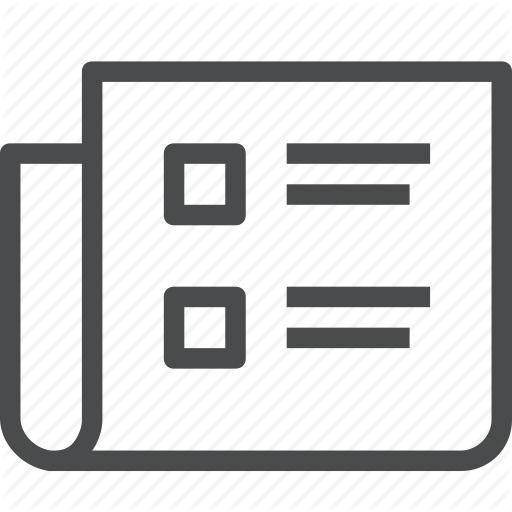
Spontaneous Formation of Altermagnetism from Orbital Ordering
V. Leeb, A. Mook, L. Smejkal, J. Knolle
Physical Review Letters 132 (23), 236701 (2024).
Altermagnetism has emerged as a third type of collinear magnetism. In contrast to standard ferromagnets and antiferromagnets, altermagnets exhibit extra even-parity wave spin order parameters resulting in a spin splitting of electronic bands in momentum space. In real space, sublattices of opposite spin polarization are anisotropic and related by rotational symmetry. In the hitherto identified altermagnetic candidate materials, the anisotropies arise from the local crystallographic symmetry. Here, we show that altermagnetism can also form as an interaction-induced electronic instability in a lattice without the crystallographic sublattice anisotropy. We provide a microscopic example of a two-orbital model showing that the coexistence of staggered antiferromagnetic and orbital order can realize robust altermagnetism. We quantify the spinsplitter conductivity as a key experimental observable and discuss material candidates for the interactioninduced realization of altermagnetism.
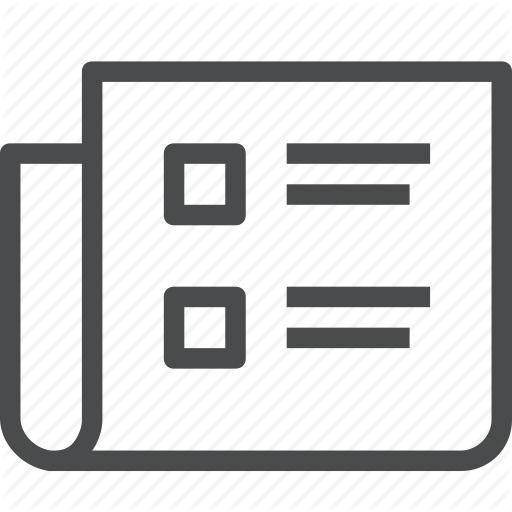
Kitaev-Heisenberg model on the star lattice: From chiral Majorana fermions to chiral triplons
P. d'Ornellas, J. Knolle
Physical Review B 109 (9), 94421 (2024).
The interplay of frustrated interactions and lattice geometry can lead to a variety of exotic quantum phases. Here we unearth a particularly rich phase diagram of the Kitaev-Heisenberg model on the star lattice, a triangle decorated honeycomb lattice breaking sublattice symmetry. In the antiferromagnetic regime, the interplay of Heisenberg coupling and geometric frustration leads to the formation of valence bond solid (VBS) phases-a singlet VBS and a bond selective triplet VBS stabilized by the Kitaev exchange. We show that the ratio of the Kitaev versus Heisenberg exchange tunes between these VBS phases and chiral quantum spin-liquid regimes. Remarkably, the VBS phases host a whole variety of chiral triplon excitations with high Chern numbers in the presence of a weak magnetic field. We discuss our results in light of a recently synthesized star lattice material and other decorated lattice systems.
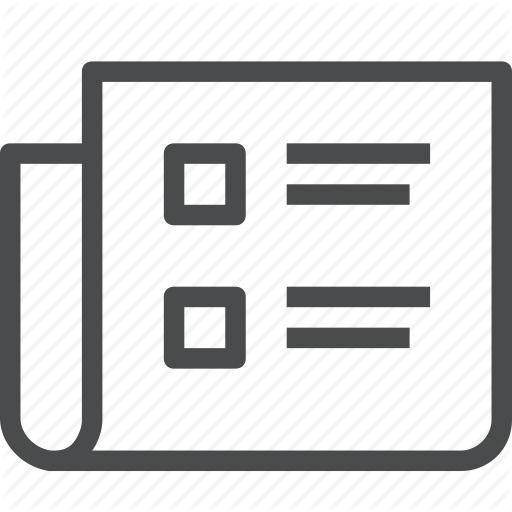
Quantum simulation of hadronic states with Rydberg-dressed atoms
Z. H. Wang, F. Y. Wang, J. Vovrosh, J. Knolle, F. Mintert, R. Mukherjee
Physical Review A 109 (3), 32613 (2024).
The phenomenon of confinement is well known in high-energy physics and can also be realized for low-energy domain-wall excitations in one-dimensional quantum spin chains. A bound state consisting of two domain walls can behave like a meson, and recently, Vovrosh et al. [PRX Quantum 3, 040309 (2022)] demonstrated that a pair of mesons could dynamically form a metastable confinement-induced bound state (consisting of four domain walls) akin to a hadronic state. However, the protocol discussed by Vovrosh et al. involving the use of interactions with characteristically nonmonotonic distance dependence is not easy to come by in nature, thus posing a challenge for its experimental realization. In this regard, Rydberg atoms can provide the required platform for simulating confinement-related physics. We exploit the flexibility offered by interacting Rydberg-dressed atoms to engineering modified spin-spin interactions for the one-dimensional transverse-field Ising model. Our numerical simulations show how Rydberg-dressed interactions can give rise to a variety of effective potentials that are suitable for hadron formation, which opens the possibility of simulating confinement physics with Rydberg platforms as a viable alternative to current trapped-ion experiments.
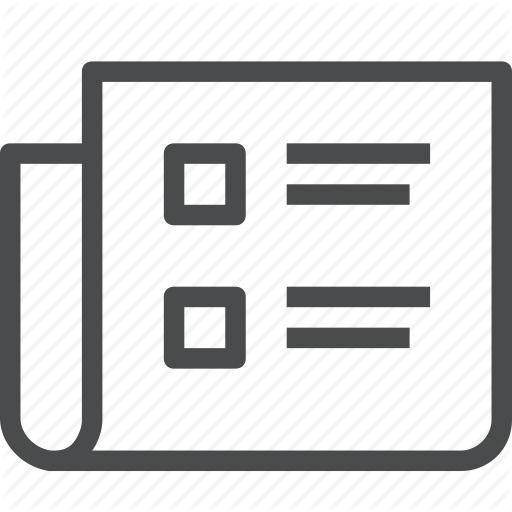
Single-hole spectra of Kitaev spin liquids: from dynamical Nagaoka ferromagnetism to spin-hole fractionalization
W. Kadow, H. K. Jin, J. Knolle, M. Knap
Npj Quantum Materials 9 (1), 32 (2024).
The dynamical response of a quantum spin liquid upon injecting a hole is a pertinent open question. In experiments, the hole spectral function, measured momentum-resolved in angle-resolved photoemission spectroscopy (ARPES) or locally in scanning tunneling microscopy (STM), can be used to identify spin liquid materials. In this study, we employ tensor network methods to simulate the time evolution of a single hole doped into the Kitaev spin-liquid ground state. Focusing on the gapped spin liquid phase, we reveal two fundamentally different scenarios. For ferromagnetic spin couplings, the spin liquid is highly susceptible to hole doping: a Nagaoka ferromagnet forms dynamically around the doped hole, even at weak coupling. By contrast, in the case of antiferromagnetic spin couplings, the hole spectrum demonstrates an intricate interplay between charge, spin, and flux degrees of freedom, best described by a parton mean-field ansatz of fractionalized holons and spinons. Moreover, we find a good agreement of our numerical results to the analytically solvable case of slow holes. Our results demonstrate that dynamical hole spectral functions provide rich information on the structure of fractionalized quantum spin liquids.
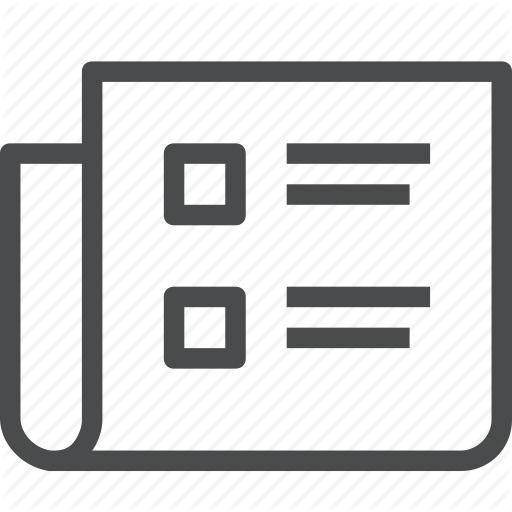
Interband scattering- and nematicity-induced quantum oscillation frequency in FeSe
V. Leeb, J. Knolle
Physical Review B 109 (8), L081109 (2024).
Understanding the nematic phase observed in the iron-chalcogenide materials is crucial for describing their superconducting pairing. Experiments on FeSe1-xSx showed that one of the slow Shubnikov-de Haas quantum oscillation frequencies disappears when tuning the material out of the nematic phase via chemical substitution or pressure, which has been interpreted as a Lifshitz transition [Coldea et al., npj Quantum Mater. 4, 2 (2019),. Reiss et al., Nat. Phys. 16, 89 (2020)]. Here, we present a generic, alternative scenario for a nematicity-induced sharp quantum oscillation frequency, which disappears in the tetragonal phase and is not connected to an underlying Fermi surface pocket. We show that different microscopic interband scattering mechanisms-for example, orbital-selective scattering-in conjunction with nematic order can give rise to this quantum oscillation frequency beyond the standard Onsager relation. We discuss implications for iron-chalcogenides and the interpretation of quantum oscillations in other correlated materials.
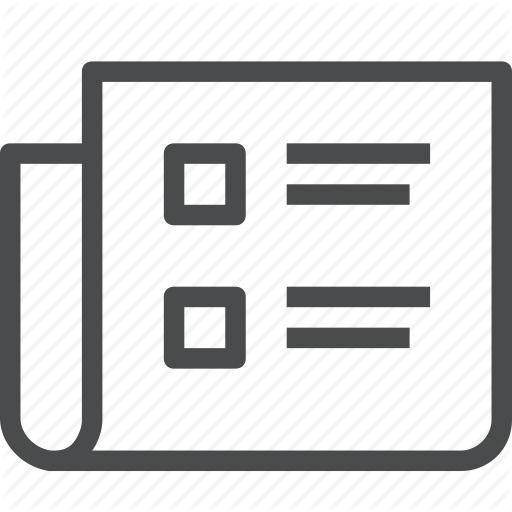
Quantum simulation of the one-dimensional Fermi-Hubbard model as a Z2 lattice-gauge theory
U. E. Khodaeva, D. L. Kovrizhin, J. Knolle
Physical Review Research 6 (1), 13032 (2024).
The Fermi-Hubbard model is one of the central paradigms in the physics of strongly correlated quantum many-body systems. Here we propose a quantum circuit algorithm based on the Z2 lattice gauge theory (LGT) representation of the one-dimensional Fermi-Hubbard model, which is suitable for implementation on current NISQ quantum computers. Within the LGT description there is an extensive number of local conserved quantities commuting with the Hamiltonian. We show how these conservation laws can be used to implement an efficient error-mitigation scheme. The latter is based on a postselection of states for noisy quantum simulators. While the LGT description requires a deeper quantum-circuit compared to a Jordan-Wigner (JW) based approach, remarkably, we find that our error-correction protocol leads to results being on par with a standard JW implementation on noisy quantum simulators.
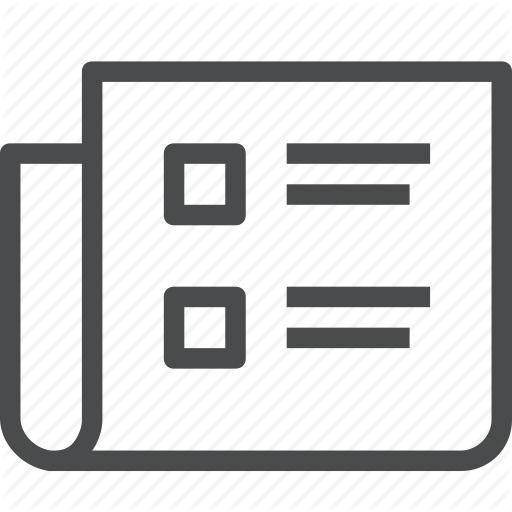
Breakdown of chiral edge modes in topological magnon insulators
J. Habel, A. Mook, J. Willsher, J. Knolle
Physical Review B 109 (2), 24441 (2024).
Topological magnon insulators (TMI) are ordered magnets supporting chiral edge magnon excitations. These edge states are envisioned to serve as topologically protected information channels in low-loss magnonic devices. The standard description of TMI is based on linear spin-wave theory (LSWT), which approximates magnons as free noninteracting particles. However, magnon excitations of TMI are genuinely interacting even at zero temperature, calling into question descriptions based on LSWT alone. Here we perform a detailed nonlinear spin-wave analysis to investigate the stability of chiral edge magnons. We identify three general breakdown mechanisms: (1) The edge magnon couples to itself, generating a finite lifetime that can be large enough to lead to a spectral annihilation of the chiral state. (2) The edge magnon hybridizes with the extended bulk magnons and, as a consequence, delocalizes away from the edge. (3) Due to a bulk-magnon mediated edge-to-edge coupling, the chiral magnons at opposite edges hybridize. We argue that, in general, these breakdown mechanisms may invalidate predictions based on LSWT and violate the notion of topological protection. We discuss strategies how the breakdown of chiral edge magnons can be avoided, e.g., via the application of large magnetic fields. Our results highlight a challenge for the realization of chiral edge states in TMI and in other bosonic topological systems without particle number conservation.
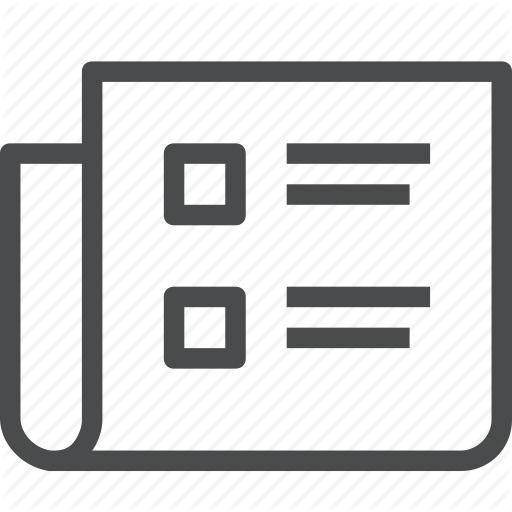
Microscopic details of two-dimensional spectroscopy of one-dimensional quantum Ising magnets
G. Sim, F. Pollmann, J. Knolle
Physical Review B 108 (13), 134423 (2023).
The identification of microscopic systems describing the low-energy properties of correlated materials has been a central goal of spectroscopic measurements. We demonstrate how two-dimensional (2D) nonlinear spectroscopy can be used to distinguish effective spin systems whose linear responses show similar behavior. Motivated by recent experiments on the quasi-1D Ising magnet CoNb2O6, we focus on two proposed systems- the ferromagnetic twisted Kitaev spin chain with bond dependent interactions and the transverse field Ising chain. The dynamical spin structure factor probed in linear response displays similar broad spectra for both systems from their fermionic domain wall excitations. In sharp contrast, the 2D nonlinear spectra of the two systems show clear qualitative differences: those of the twisted Kitaev spin chain contain off-diagonal peaks originating from the bond dependent interactions and transitions between different fermion bands absent in the transverse field Ising chain. We discuss the different signatures of spin fractionalization in integrable and nonintegrable regimes of the systems and their connection to experiments.
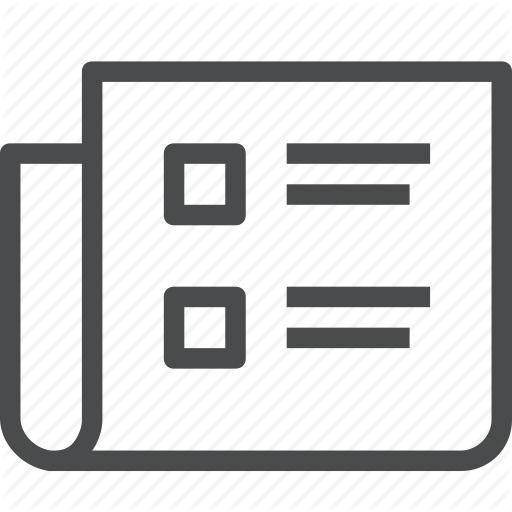
An exact chiral amorphous spin liquid
G. Cassella, P. d'Ornellas, T. Hodson, W. M. H. Natori, J. Knolle
Nature Communications 14 (1), 6663 (2023).
Topological insulator phases of non-interacting particles have been generalized from periodic crystals to amorphous lattices, which raises the question whether topologically ordered quantum many-body phases may similarly exist in amorphous systems? Here we construct a soluble chiral amorphous quantum spin liquid by extending the Kitaev honeycomb model to random lattices with fixed coordination number three. The model retains its exact solubility but the presence of plaquettes with an odd number of sides leads to a spontaneous breaking of time reversal symmetry. We unearth a rich phase diagram displaying Abelian as well as a non-Abelian quantum spin liquid phases with a remarkably simple ground state flux pattern. Furthermore, we show that the system undergoes a finite-temperature phase transition to a conducting thermal metal state and discuss possible experimental realisations. Recently topological phases have been generalized to amorphous materials, but demonstrations have been limited to non-interacting particles. Cassella et al. show the emergence of chiral amorphous quantum spin liquid in an exactly soluble model by extending the Kitaev honeycomb model to random lattices.
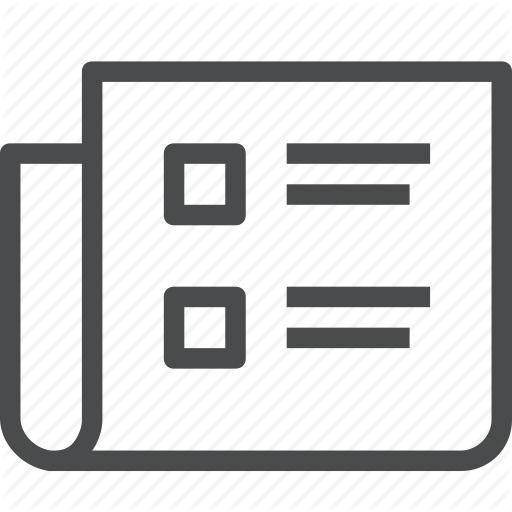
Quantum oscillations of the quasiparticle lifetime in a metal
N. Huber, V. Leeb, A. Bauer, G. Benka, J. Knolle, C. Pfleiderer, M. A. Wilde
Nature 621 (7978), 276-+ (2023).
Following nearly a century of research, it remains a puzzle that the low-lying excitations of metals are remarkably well explained by effective single-particle theories of non-interacting bands(1-4). The abundance of interactions in real materials raises the question of direct spectroscopic signatures of phenomena beyond effective single-particle, single-band behaviour. Here we report the identification of quantum oscillations (QOs) in the three-dimensional topological semimetal CoSi, which defy the standard description in two fundamental aspects. First, the oscillation frequency corresponds to the difference of semiclassical quasiparticle (QP) orbits of two bands, which are forbidden as half of the trajectory would oppose the Lorentz force. Second, the oscillations exist up to above 50 K, in strong contrast to all other oscillatory components, which vanish below a few kelvin. Our findings are in excellent agreement with generic model calculations of QOs of the QP lifetime (QPL). Because the only precondition for their existence is a nonlinear coupling of at least two electronic orbits, for example, owing to QP scattering on defects or collective excitations, such QOs of the QPL are generic for any metal featuring Landau quantization with several orbits. They are consistent with certain frequencies in topological semimetals(5-9), unconventional superconductors(10,11), rare-earth compounds(12-14) and Rashba systems(15), and permit to identify and gauge correlation phenomena, for example, in two-dimensional materials(16,17) and multiband metals(18).
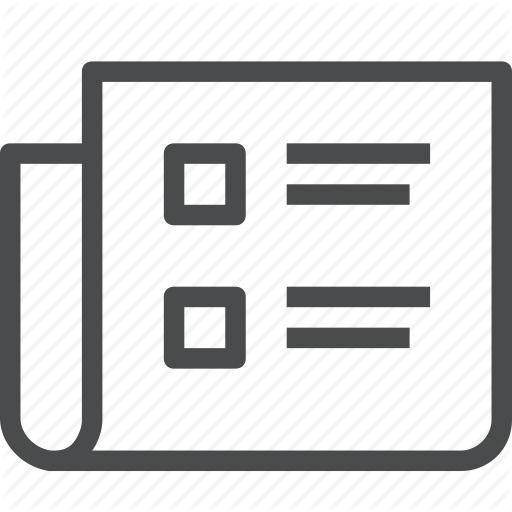
Temporal disorder in spatiotemporal order
H. Z. Zhao, J. Knolle, R. Moessner
Physical Review B 108 (10), L100203 (2023).
Time-dependent driving holds the promise of realizing dynamical phenomena absent in static systems. Here, we introduce a correlated random driving protocol to realize a spatiotemporal order that cannot be achieved even by periodic driving, thereby extending the discussion of time translation symmetry breaking to randomly driven systems. We find a combination of temporally disordered micromotion with prethermal stroboscopic spatiotemporal long-range order. This spatiotemporal order remains robust against generic perturbations, with an algebraically long prethermal lifetime where the scaling exponent strongly depends on the symmetry of the perturbation, which we account for analytically.
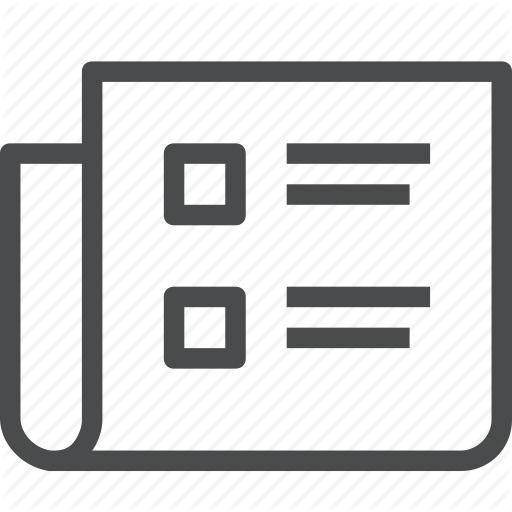
Theory of difference-frequency quantum oscillations
V. Leeb, J. Knolle
Physical Review B 108 (5), 54202 (2023).
Quantum oscillations (QOs) describe the periodic variation of physical observables as a function of inverse magnetic field in metals. The Onsager relation connects the basic QO frequencies with the extremal areas of closed Fermi surface pockets, and the theory of magnetic breakdown explains the observation of sums of QO frequencies at high magnetic fields. Here we develop a quantitative theory of difference-frequency QOs in two and three-dimensional metals with multiple Fermi pockets with parabolic or linearly dispersing excitations. We show that a nonlinear interband coupling, e.g., in the form of interband impurity scattering, can give rise to otherwise forbidden QO frequencies which can persist to much higher temperatures compared to the basis frequencies. We discuss the experimental implications of our findings for various material candidates, for example multifold fermion systems, like CoSi, and the relation to magneto-intersubband oscillations known for coupled two-dimensional electron gases.
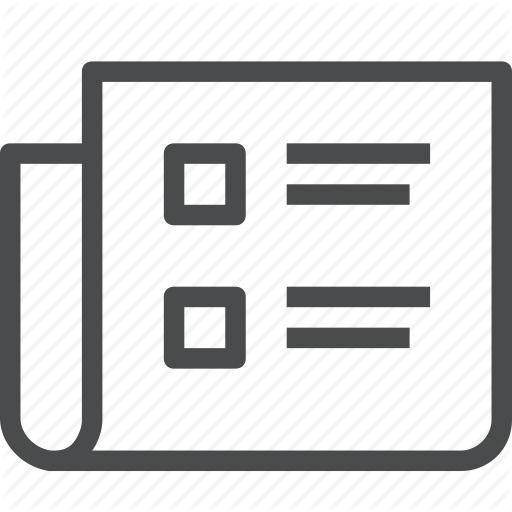
Fractionalized Prethermalization in a Driven Quantum Spin Liquid
H. K. Jin, J. Knolle, M. Knap
Physical Review Letters 130 (22), 226701 (2023).
Quantum spin liquids subject to a periodic drive can display fascinating nonequilibrium heating behavior because of their emergent fractionalized quasiparticles. Here, we investigate a driven Kitaev honeycomb model and examine the dynamics of emergent Majorana matter and Z2 flux excitations. We uncover a distinct two-step heating profile-dubbed fractionalized prethermalization-and a quasistationary state with vastly different temperatures for the matter and the flux sectors. We argue that this peculiar prethermalization behavior is a consequence of fractionalization. Furthermore, we discuss an exper-imentally feasible protocol for preparing a zero-flux initial state of the Kiteav honeycomb model with a low energy density, which can be used to observe fractionalized prethermalization in quantum information processing platforms.
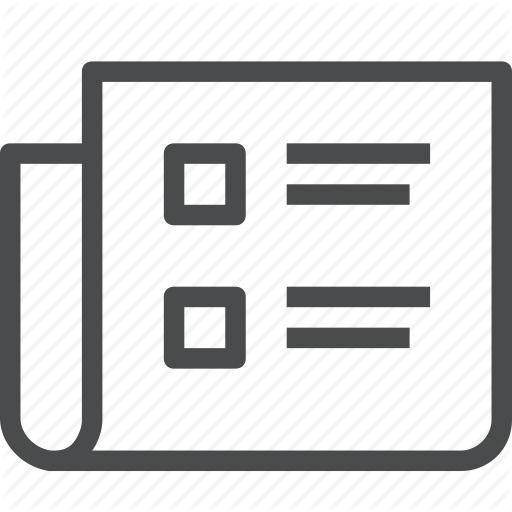
Twisting the Dirac cones of the SU(4) spin-orbital liquid on the honeycomb lattice
H. K. Jin, W. M. H. Natori, J. Knolle
Physical Review B 107 (18), L180401 (2023).
By combining the density matrix renormalization group (DMRG) method with Gutzwiller projected wave functions, we study the SU(4) symmetric spin-orbital model on the honeycomb lattice. We find that the ground states can be well described by a Gutzwiller projected pi-flux state with Dirac-type gapless excitations at one quarter filling. Although these Dirac points are gapped by emergent gauge fluxes on finite cylinders, they govern the critical behavior in the thermodynamic limit. By inserting a theta = pi spin flux to twist the boundary condition, we can shift the gapless sector to the ground state, which provides compelling evidence for the presence of a gapless Dirac spin-orbital liquid.
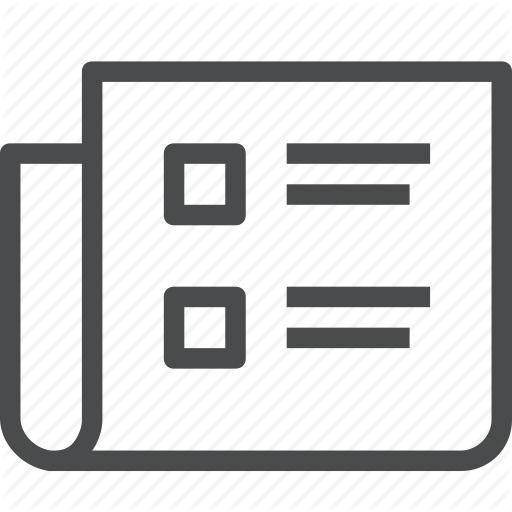
Nonlinear spectroscopy of bound states in perturbed Ising spin chains
G. Sim, J. Knolle, F. Pollmann
Physical Review B 107 (10), L100404 (2023).
We study the nonlinear response of nonintegrable one-dimensional (1D) spin models using infinite matrix-product state techniques. As a benchmark and demonstration of the method, we first calculate the two-dimensional (2D) coherent spectroscopy for the exactly soluble ferromagnetic transverse field Ising model where excitations are freely moving domain walls. We then investigate the distinct signatures of confined bound states by introducing a longitudinal field and observe the emergence of strong nonrephasinglike signals. To interpret the observed phenomena, we use a two-kink approximation to perturbatively compute the 2D spectra. We find good agreement in comparison with the exact results of the infinite matrix-product state method in the strongly confined regime. We discuss the relevance of our results for quasi-1D Ising spin chain materials, such as CoNb2O6.
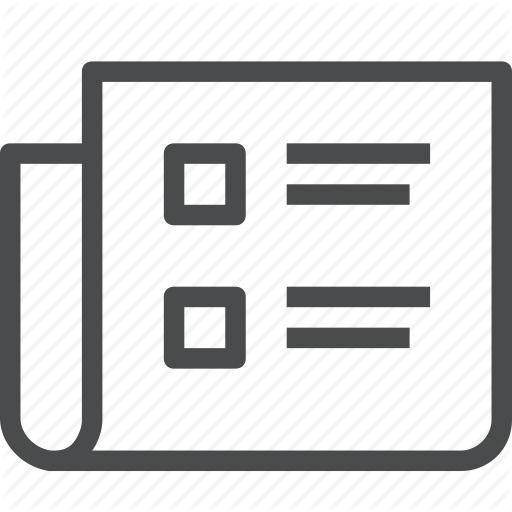
Magnetic excitations, phase diagram, and order-by-disorder in the extended triangular-lattice Hubbard model
J. Willsher, H. K. Jin, J. Knolle
Physical Review B 107 (6), 64425 (2023).
The dynamical structure factor is an important observable of quantum magnets but due to numerical and theoretical limitations, it remains a challenge to make predictions for Hubbard-like models beyond one di-mension. In this work, we study the magnetic excitations of the triangular lattice Hubbard model including next-nearest-neighbor hopping. Starting from the expected 120 degrees and stripe magnetic orders, we compute the magnon spectra within a self-consistent random phase approximation. In the stripe phase, we generically find accidental zero modes related to a classical degeneracy known from the corresponding J1 -J2 Heisenberg model. We extend the order-by-disorder mechanism to Hubbard systems and show how quantum fluctuations stabilize the stripe order. In addition, the frustration-induced condensation of magnon modes allows us to map out the entire phase diagram which is in remarkable agreement with recent numerical works. We discuss connections to experiments on triangular lattice compounds and the relation of our results to the proposed chiral spin liquid phase.
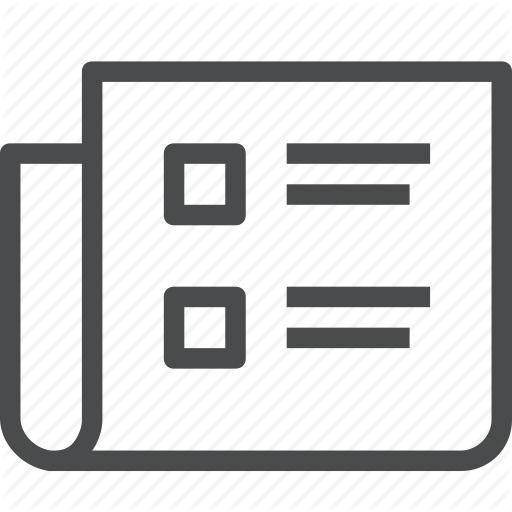
Bridging the gap between classical and quantum many-body information dynamics
A. Pizzi, D. Malz, A. Nunnenkamp, J. Knolle
Physical Review B 106 (21), 214303 (2022).
"The fundamental question of how information spreads in closed quantum many-body systems is often addressed through the lens of the bipartite entanglement entropy, a quantity that describes correlations in a comprehensive (nonlocal) way. Among the most striking features of the entanglement entropy are its unbounded linear growth in the thermodynamic limit, its asymptotic extensivity in finite-size systems, and the possibility of measurement-induced phase transitions, all of which have no obvious classical counterpart. Here, we show how these key qualitative features emerge naturally also in classical information spreading, as long as one treats the classical many-body problem on par with the quantum one, that is, by explicitly accounting for the exponentially large classical probability distribution. Our analysis is supported by extensive numerics on prototypical cellular automata and Hamiltonian systems, for which we focus on the classical mutual information and also introduce a ""classical entanglement entropy."" Our study sheds light on the nature of information spreading in classical and quantum systems, and opens avenues for quantum-inspired classical approaches across physics, information theory, and statistics."
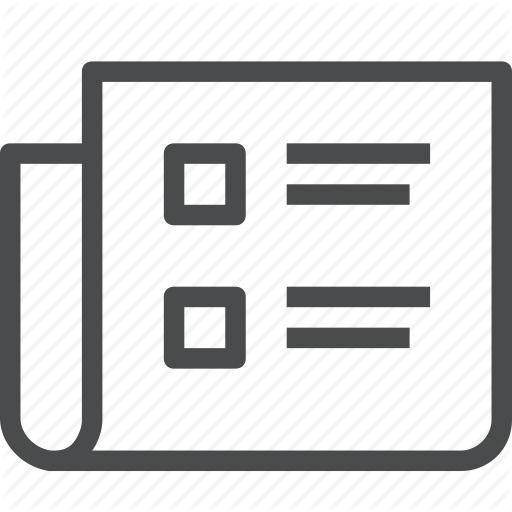
Thermal spin dynamics of Kitaev magnets: Scattering continua and magnetic field induced phases within a stochastic semiclassical approach
O. Franke, D. Calugaru, A. Nunnenkamp, J. Knolle
Physical Review B 106 (17), 174428 (2022).
"The honeycomb magnet a-RuCl3 is a prime candidate material for realizing the Kitaev quantum spin liquid (QSL), but it shows long-range magnetic order at low temperature. Nevertheless, its broad inelastic neutron scattering (INS) response at finite frequency has been interpreted as that of a ""proximate QSL."" A moderate in-plane magnetic field indeed melts the residual zigzag order, giving rise to peculiar intermediate-field phases before the high-field polarized state. In INS measurements the low-frequency spin waves disappear, leading to a broad scattering continuum in the field-induced intermediate regime, whose nature is currently under debate. Here, we study the magnetic-field-dependent spin dynamics of the K -F -F' model within a stochastic semiclassical treatment, which incorporates the effect of finite-temperature fluctuations. At temperatures relevant for INS experiments, we show how the excitations of the zigzag phase broaden and that the different intermediate phases all show a similar continuum response. We discuss the implications of our results for experiments and highlight the importance of distinguishing finite-temperature fluctuations from genuine quantum fractionaliza-tion signatures in frustrated magnets."
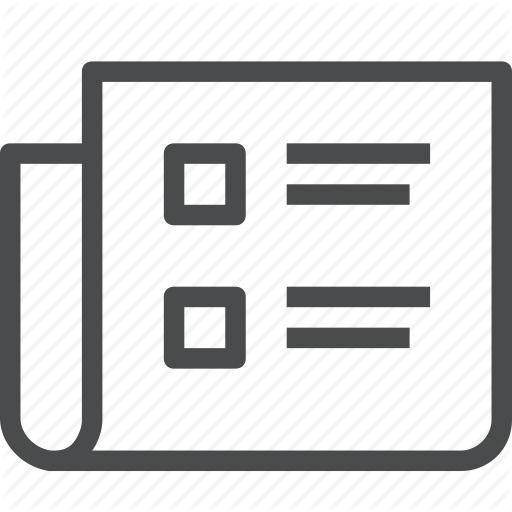
Disorder-free localization with Stark gauge protection
H. F. Lang, P. Hauke, J. Knolle, F. Grusdt, J. C. Halimeh
Physical Review B 106 (17), 174305 (2022).
Disorder-free localization in translation-invariant gauge theories presents a counterintuitive yet powerful framework of ergodicity breaking in quantum many-body physics. The fragility of this phenomenon in the presence of gauge-breaking errors has recently been addressed, but no scheme has been able to reliably stabilize disorder-free localization through all accessible evolution times while preserving the disorder-free property. Here, we introduce the concept of Stark gauge protection, which entails a linear sum in gauge-symmetry local (pseudo)generators weighted by a Stark potential. Using exact diagonalization and Krylov-based methods, we show how this scheme can stabilize or even enhance disorder-free localization against gauge-breaking errors in U(1) and Z2 gauge theories up to all accessible evolution times, without inducing bona fide Stark many-body localization. We show through a Magnus expansion that the dynamics under Stark gauge protection is described by an effective Hamiltonian where gauge-breaking terms are suppressed locally by the protection strength and additionally by the matter site index, which we argue is the main reason behind stabilizing the localization up to all accessible times. Our scheme is readily feasible in modern ultracold-atom experiments and Rydberg-atom setups with optical tweezers.
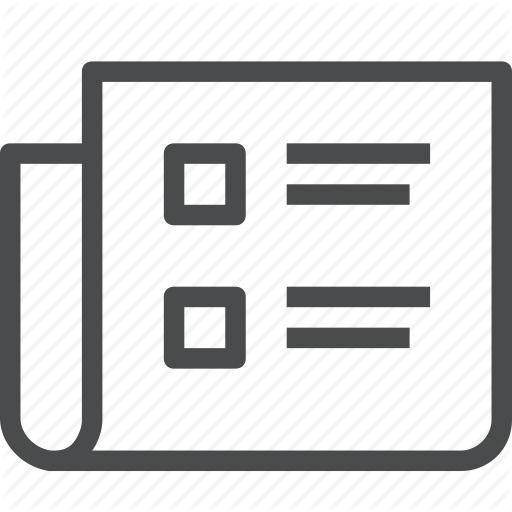
Prethermal nematic order and staircase heating in a driven frustrated Ising magnet with dipolar interactions
H. K. Jin, A. Pizzi, J. Knolle
Physical Review B 106 (14), 144312 (2022).
Many-body systems subject to a high-frequency drive can show intriguing thermalization behavior. Prior to heating to a featureless infinite-temperature state, these systems can spend an exponentially long time in prethermal phases characterized by various kinds of order. Here, we uncover the rich nonequilibrium phase diagram of a driven frustrated two-dimensional Ising magnet with competing short-range ferromagnetic and long-range dipolar interactions. We show that the ordered stripe and nematic phases, which appear in equilibrium as a function of temperature, underpin subsequent prethermal phases in a new multistep heating process en route towards the ultimate heat death. We discuss implications for experiments on ferromagnetic thin films and other driving induced phenomena in frustrated magnets.
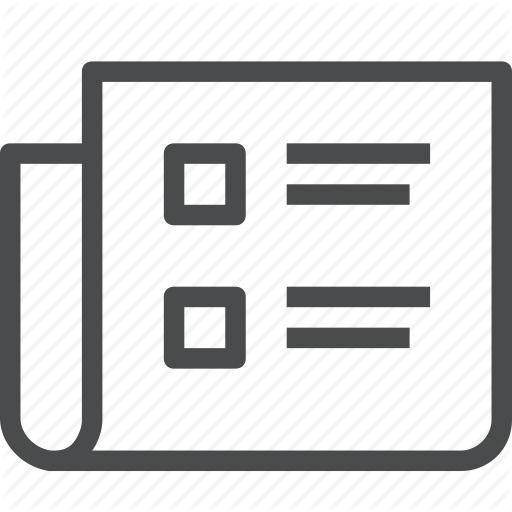
Dynamical Hadron Formation in Long-Range Interacting Quantum Spin Chains
J. Vovrosh, R. Mukherjee, A. Bastianello, J. Knolle
Prx Quantum 3 (4), 40309 (2022).
The study of confinement in quantum spin chains has seen a large surge of interest in recent years. It is not only important for understanding a range of effective one-dimensional condensed-matter realiza-tions but it also shares some of the nonperturbative physics with quantum chromodynamics (QCD), which makes it a prime target for current quantum simulation efforts. In analogy to QCD, the confinement -induced two-particle bound states that appear in these models are dubbed mesons. Here, we study scattering events due to meson collisions in a quantum spin chain with long-range interactions such that two mesons have an extended interaction. We show how novel hadronic bound states, e.g., with four con-stituent particles akin to tetraquarks, may form dynamically in fusion events. In a natural collision their signal is weak, as elastic meson scattering dominates. However, we propose two controllable protocols that allow for a clear observation of dynamical hadron formation. We discuss how this physics can be simulated in trapped-ion or Rydberg-atom setups.
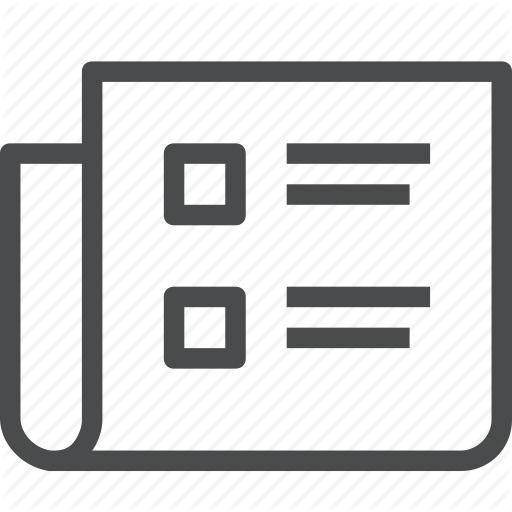
Suppression of Interband Heating for Random Driving
H. Z. Zhao, J. Knolle, R. Moessner, F. Mintert
Physical Review Letters 129 (12), 120605 (2022).
Heating to high-lying states strongly limits the experimental observation of driving induced non -equilibrium phenomena, particularly when the drive has a broad spectrum. Here we show that, for entire families of structured random drives known as random multipolar drives, particle excitation to higher bands can be well controlled even away from a high-frequency driving regime. This opens a window for observing drive-induced phenomena in a long-lived prethermal regime in the lowest band.
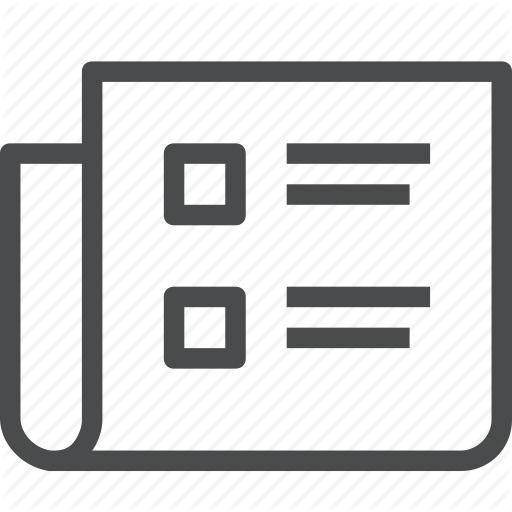
Unveiling the S=3/2 Kitaev honeycomb spin liquids
H. K. Jin, W. M. H. Natori, F. Pollmann, J. Knolle
Nature Communications 13 (1), 3813 (2022).
Recently, material realizations of the spin 3/2 Kitaev honeycomb model have been proposed, but the model has not been solved by either analytical or numerical methods. Here the authors report exact results for the spin 3/2 model consistent with numerical simulations, and find gapped and gapless quantum spin liquids. The S=3/2 Kitaev honeycomb model (KHM) is a quantum spin liquid (QSL) state coupled to a static Z(2) gauge field. Employing an SO(6) Majorana representation of spin3/2's, we find an exact representation of the conserved plaquette fluxes in terms of static Z(2) gauge fields akin to the S=1/2 KHM which enables us to treat the remaining interacting matter fermion sector in a parton mean-field theory. We uncover a ground-state phase diagram consisting of gapped and gapless QSLs. Our parton description is in quantitative agreement with numerical simulations, and is furthermore corroborated by the addition of a [001] single ion anisotropy (SIA) which continuously connects the gapless Dirac QSL of our model with that of the S=1/2 KHM. In the presence of a weak [111] SIA, we discuss an emergent chiral QSL within a perturbation theory.
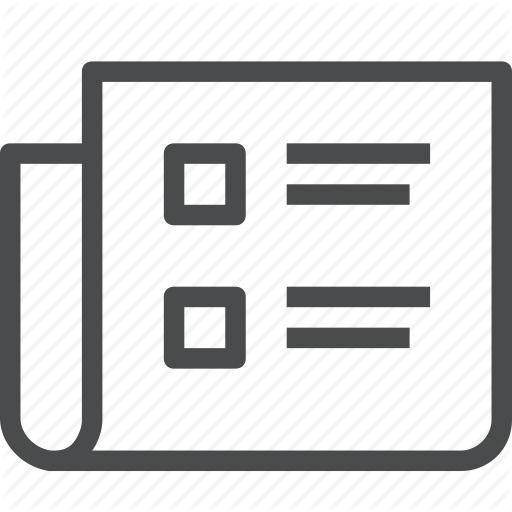
Measurement-induced phase transition in a chaotic classical many-body system
J. Willsher, S. W. Liu, R. Moessner, J. Knolle
Physical Review B 106 (2), 24305 (2022).
Local measurements in quantum systems are projective operations which act to counteract the spread of quan-tum entanglement. Recent work has shown that local, random measurements applied to a generic volume-law entanglement generating many-body system are able to force a transition into an area-law phase. This work shows that projective operations can also force a similar classical phase transition,. we show that local projections in a chaotic system can freeze information dynamics. In rough analogy with measurement-induced phase transitions, this is characterized by an absence of information spreading instead of entanglement entropy. We leverage a damage-spreading model of the classical transition to predict the butterfly velocity of the system both near to and away from the transition point. We map out the full phase diagram and show that the critical point is shifted by local projections, but remains in the directed percolation universality class. We discuss the implication for other classical chaotic many-body systems and the relation to synchronization transitions.
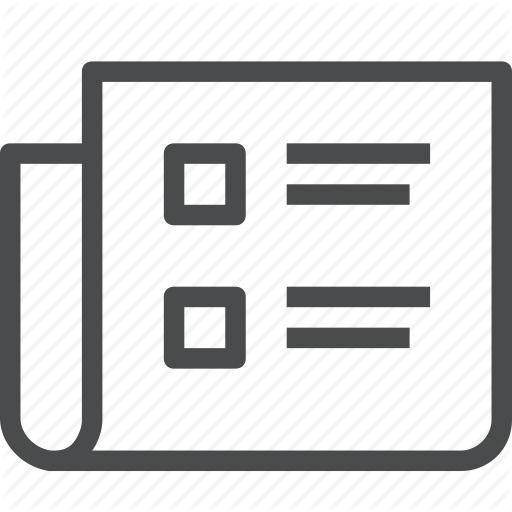
Berry curvature-induced local spin polarisation in gated graphene/WTe2 heterostructures
L. Powalla, J. Kiemle, E. J. Konig, A. P. Schnyder, J. Knolle, K. Kern, A. Holleitner, C. Kastl, M. Burghard
Nature Communications 13 (1), 3152 (2022).
Experimental control of local spin-charge interconversion is of primary interest for spintronics. Van der Waals (vdW) heterostructures combining graphene with a strongly spin-orbit coupled two-dimensional (2D) material enable such functionality by design. Electric spin valve experiments have thus far provided global information on such devices, while leaving the local interplay between symmetry breaking, charge flow across the heterointerface and aspects of topology unexplored. Here, we probe the gate-tunable local spin polarisation in current-driven graphene/WTe2 heterostructures through magneto-optical Kerr microscopy. Even for a nominal in-plane transport, substantial out-of-plane spin accumulation is induced by a corresponding out-of-plane current flow. We present a theoretical model which fully explains the gate- and bias-dependent onset and spatial distribution of the intense Kerr signal as a result of a non-linear anomalous Hall effect in the heterostructure, which is enabled by its reduced point group symmetry. Our findings unravel the potential of 2D heterostructure engineering for harnessing topological phenomena for spintronics, and constitute an important step toward nanoscale, electrical spin control. Spin-based electronics offers significantly improved efficiency, but a major challenge is the electric manipulation of spin. Here, Powalla et al find a large gate induced spinpolarization in graphene/WTe2 heterostructures, illustrating the potential of such heterostructures for spintronics.
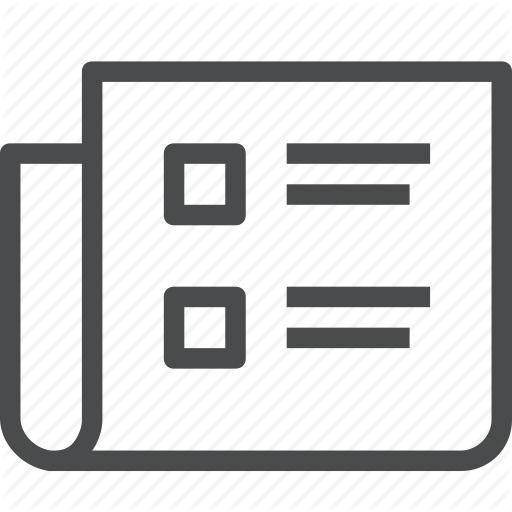
Localization persisting under aperiodic driving
H. Z. Zhao, F. Mintert, J. Knolle, R. Moessner
Physical Review B 105 (22), L220202 (2022).
"Localization may survive in periodically driven (Floquet) quantum systems, but is generally unstable for aperiodic drives. In this Letter, we identify a hidden conservation law originating from a chiral symmetry in a disordered spin-21 XX chain. This protects indefinitely long-lived localization for general-even aperiodic-drives. Therefore, rather counterintuitively, adding further potential disorder which spoils the conservation law delocalizes the system, via a controllable parametrically long-lived prethermal regime. This provides an example of persistent single-particle ""localization without eigenstates."""
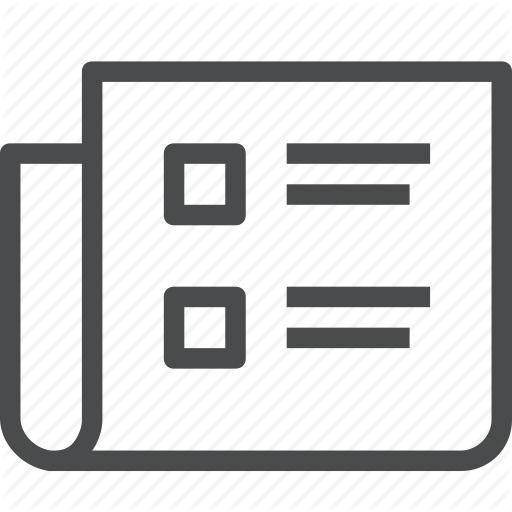
Anomalous random multipolar driven insulators
H. Z. Zhao, M. S. Rudner, R. Moessner, J. Knolle
Physical Review B 105 (24), 245119 (2022).
It is by now well established that periodically driven quantum many-body systems can realize topological nonequilibrium phases without any equilibrium counterpart. Here we show that, even in the absence of time translation symmetry, nonequilibrium topological phases of matter can exist in aperiodically driven systems for tunably parametrically long prethermal lifetimes. As a prerequisite, we first demonstrate the existence of longlived prethermal Anderson localization in two dimensions under random multipolar driving. We then show that the localization may be topologically nontrivial with a quantized bulk orbital magnetization even though there are no well-defined Floquet operators. We further confirm the existence of this anomalous random multipolar driven insulator by detecting quantized charge pumping at the boundaries, which renders it experimentally observable.
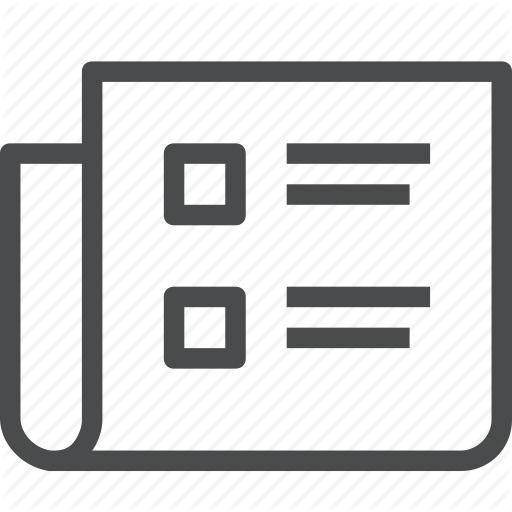
Enhancing Disorder-Free Localization through Dynamically Emergent Local Symmetries
J. C. Halimeh, L. Homeier, H. Z. Zhao, A. Bohrdt, F. Grusdt, P. Hauke, J. Knolle
Prx Quantum 3 (2), 19 (2022).
Disorder-free localization is a recently discovered phenomenon of nonergodicity that can emerge in quantum many-body systems hosting gauge symmetries when the initial state is prepared in a superposition of gauge superselection sectors. Thermalization is then prevented up to all accessible evolution times despite the model being nonintegrable and translation invariant. In a recent work [Halimeh et al., arXiv:2111.02427 (2021)], it has been shown that terms linear in the gauge-symmetry generator stabilize disorder-free localization in U(1) gauge theories against gauge errors that couple different superselection sectors. Here, we show in the case of Z2 gauge theories that disorder-free localization can not only be stabilized, but also enhanced by the addition of translation-invariant terms linear in a local Z2 pseudogenerator that acts identically to the full generator in a single superselection sector, but not necessarily outside of it. We show analytically and numerically how this leads through the quantum Zeno effect to the dynamical emergence of a renormalized gauge theory with an enhanced local symmetry, which contains the Z2 gauge symmetry of the ideal model, associated with the Z2 pseudogenerator. The resulting proliferation of superselection sectors due to this dynamically emergent gauge theory creates an effective disorder greater than that in the original model, thereby enhancing disorder-free localization. We demonstrate the experimental feasibility of the Z2 pseudogenerator by providing a detailed readily implementable experimental proposal for the observation of disorder-free localization in a Rydberg setup.
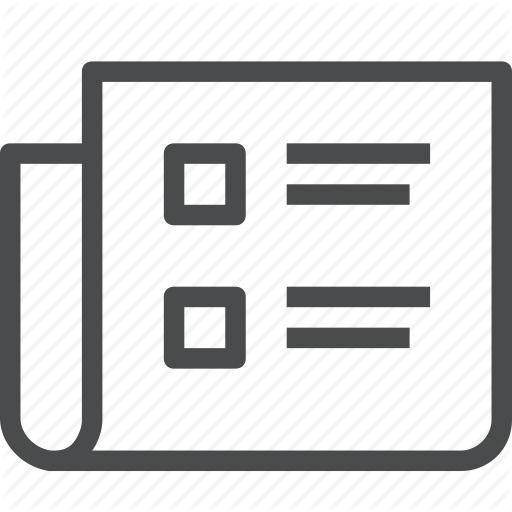
Optical phonons coupled to a Kitaev spin liquid
A. Metavitsiadis, W. Natori, J. Knolle, W. Brenig
Physical Review B 105 (16), 165151 (2022).
Emergent excitation continua in frustrated magnets are a fingerprint of fractionalization, characteristic of quantum spin-liquid states. Recent evidence from Raman scattering for a coupling between such continua and lattice degrees of freedom in putative Kitaev magnets may provide insight into the nature of the fractionalized quasiparticles. Here we study the renormalization of optical phonons coupled to the underlying Z2 quantum spin liquid. We show that phonon line shapes acquire an asymmetry, observable in light scattering and originating from two distinct sources, namely, the dispersion of the Majorana continuum and the Fano effect. Moreover, we find that the phonon lifetimes increase with increasing temperature due to thermal blocking of the available phase space. Finally, in contrast to low-energy probes, optical phonon renormalization is rather insensitive to thermally excited gauge fluxes and barely susceptible to external magnetic fields.
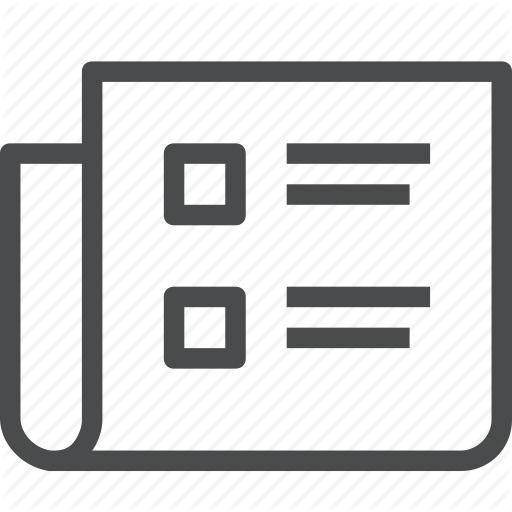
Confinement-induced impurity states in spin chains
J. Vovrosh, H. Z. Zhao, J. Knolle, A. Bastianello
Physical Review B 105 (10), L100301 (2022).
Quantum simulators hold the promise of probing central questions of high-energy physics in tunable condensed matter platforms, for instance, the physics of confinement. Local defects can be an obstacle in these setups, harming their simulation capabilities. However, defects in the form of impurities can also be useful as probes of many-body correlations and may lead to fascinating new phenomena themselves. Here, we investigate the interplay between impurity and confinement physics in a basic spin chain setup, showing the emergence of exotic excitations as impurity-meson bound states with a long lifetime. For weak confinement, semiclassical approximations can describe the capture process in a meson-impurity scattering event. In the strong-confining regime, intrinsic quantum effects are visible through the quantization of the emergent bound state energies which can be in simulators.
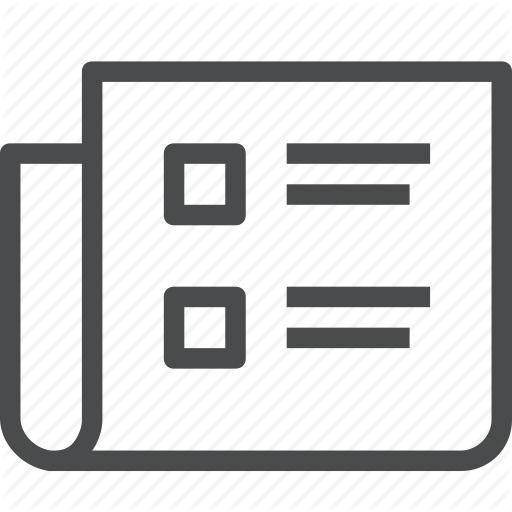
Orthogonal Quantum Many-Body Scars
H. Z. Zhao, A. Smith, F. Mintert, J. Knolle
Physical Review Letters 127 (15), 150601 (2021).
Quantum many-body scars have been put forward as counterexamples to the eigenstate thermalization hypothesis. These atypical states are observed in a range of correlated models as long-lived oscillations of local observables in quench experiments starting from selected initial states. The long-time memory is a manifestation of quantum nonergodicity generally linked to a subextensive generation of entanglement entropy, the latter of which is widely used as a diagnostic for identifying quantum many-body scars numerically as low entanglement outliers. Here we show that by adding kinetic constraints to a fractionalized orthogonal metal, we can construct a minimal model with orthogonal quantum many-body scars leading to persistent oscillations with infinite lifetime coexisting with rapid volume-law entanglement generation. Our example provides new insights into the link between quantum ergodicity and many-body entanglement while opening new avenues for exotic nonequilibrium dynamics in strongly correlated multicomponent quantum systems.
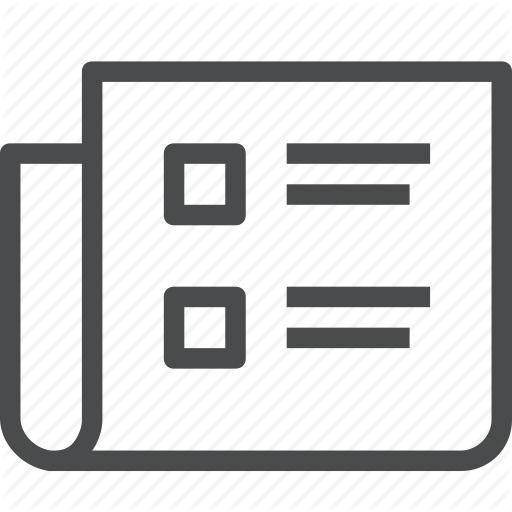
Classical approaches to prethermal discrete time crystals in one, two, and three dimensions
A. Pizzi, A. Nunnenkamp, J. Knolle
Physical Review B 104 (9), 94308 (2021).
We provide a comprehensive account of prethermal discrete time crystals within classical Hamiltonian dynamics, complementing and extending our recent work [A. Pizzi, A. Nunnenkamp, and J. Knolle, Phys. Rev. Lett. 127, 140602 (2021)]. Considering power-law interacting spins on one-, two-, and three-dimensional hypercubic lattices, we investigate the interplay between dimensionality and interaction range in the stabilization of these nonequilibrium phases of matter that break the discrete time-translational symmetry of a periodic drive.
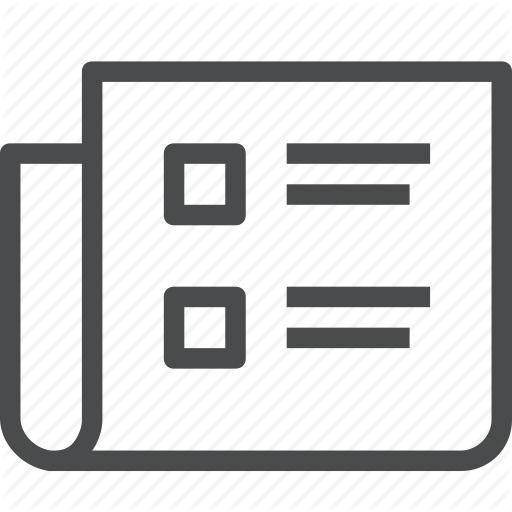
Simple mitigation of global depolarizing errors in quantum simulations
J. Vovrosh, K. E. Khosla, S. Greenaway, C. Self, M. S. Kim, J. Knolle
Physical Review E 104 (3), 35309 (2021).
To get the best possible results from current quantum devices error mitigation is essential. In this work we present a simple but effective error mitigation technique based on the assumption that noise in a deep quantum circuit is well described by global depolarizing error channels. By measuring the errors directly on the device, we use an error model ansatz to infer error-free results from noisy data. We highlight the effectiveness of our mitigation via two examples of recent interest in quantum many-body physics: entanglement measurements and real-time dynamics of confinement in quantum spin chains. Our technique enables us to get quantitative results from the IBM quantum computers showing signatures of confinement, i.e., we are able to extract the meson masses of the confined excitations which were previously out of reach. Additionally, we show the applicability of this mitigation protocol in a wider setting with numerical simulations of more general tasks using a realistic error model. Our protocol is device-independent, simply implementable, and leads to large improvements in results if the global errors are well described by depolarization.
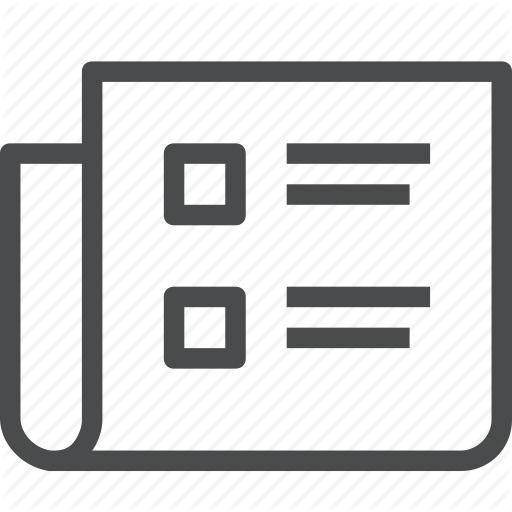
Classical Prethermal Phases of Matter
A. Pizzi, A. Nunnenkamp, J. Knolle
Physical Review Letters 127 (14), 140602 (2021).
Systems subject to a high-frequency drive can spend an exponentially long time in a prethermal regime, in which novel phases of matter with no equilibrium counterpart can be realized. Because of the notorious computational challenges of quantum many-body systems, numerical investigations in this direction have remained limited to one spatial dimension, in which long-range interactions have been proven a necessity. Here, we show that prethermal nonequilibrium phases of matter are not restricted to the quantum domain. Studying the Hamiltonian dynamics of a large three-dimensional lattice of classical spins, we provide the first numerical proof of prethermal phases of matter in a system with short-range interactions. Concretely, we find higher-order as well as fractional discrete time crystals breaking the time-translational symmetry of the drive with unexpectedly large integer as well as fractional periods. Our work paves the way toward the exploration of novel prethermal phenomena by means of classical Hamiltonian dynamics with virtually no limitations on the system's geometry or size, and thus with direct implications for experiments.
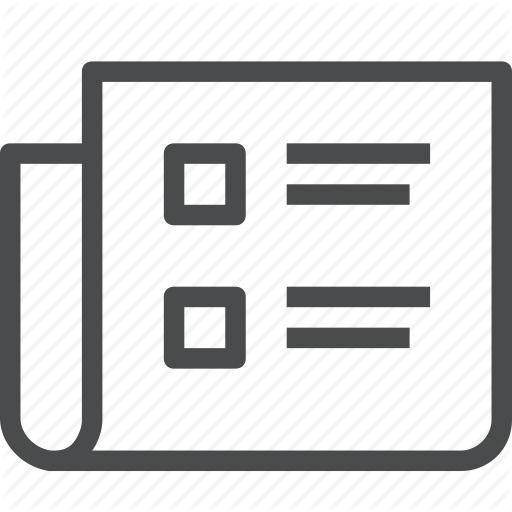
Variational quantum algorithm with information sharing
C. N. Self, K. E. Khosla, A. W. R. Smith, F. Sauvage, P. D. Haynes, J. Knolle, F. Mintert, M. S. Kim
Npj Quantum Information 7 (1), 116 (2021).
We introduce an optimisation method for variational quantum algorithms and experimentally demonstrate a 100-fold improvement in efficiency compared to naive implementations. The effectiveness of our approach is shown by obtaining multi-dimensional energy surfaces for small molecules and a spin model. Our method solves related variational problems in parallel by exploiting the global nature of Bayesian optimisation and sharing information between different optimisers. Parallelisation makes our method ideally suited to the next generation of variational problems with many physical degrees of freedom. This addresses a key challenge in scaling-up quantum algorithms towards demonstrating quantum advantage for problems of real-world interest.
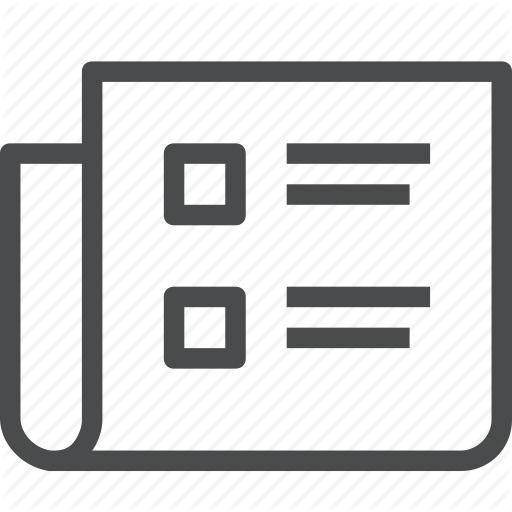
Rigorous Bounds on the Heating Rate in Thue-Morse Quasiperiodically and Randomly Driven Quantum Many-Body Systems
T. Mori, H. Z. Zhao, F. Mintert, J. Knolle, R. Moessner
Physical Review Letters 127 (5), 50602 (2021).
The nonequilibrium quantum dynamics of closed many-body systems is a rich yet challenging field. While recent progress for periodically driven (Floquet) systems has yielded a number of rigorous results, our understanding on quantum many-body systems driven by rapidly varying but aperiodic and quasiperiodic driving is still limited. Here, we derive rigorous, nonperturbative, bounds on the heating rate in quantum many-body systems under Thue-Morse quasiperiodic driving and under random multipolar driving, the latter being a tunably randomized variant of the former. In the process, we derive a static effective Hamiltonian that describes the transient prethermal state, including the dynamics of local observables. Our bound for Thue-Morse quasiperiodic driving suggests that the heating time scales like (omega/g)(-C) (ln()(omega/)(g)) with a positive constant C and a typical energy scale g of the Hamiltonian, in agreement with our numerical simulations.
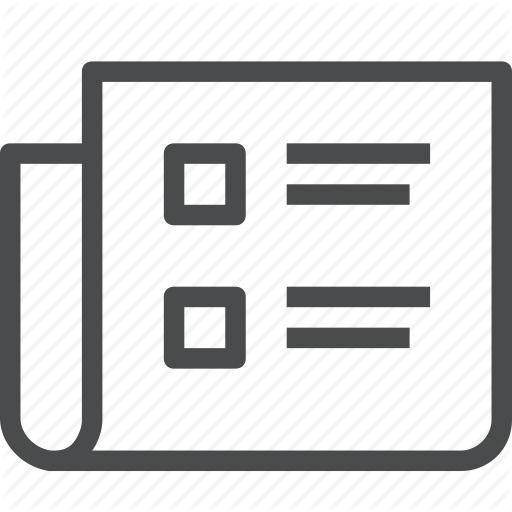
One-dimensional long-range Falikov-Kimball model: Thermal phase transition and disorder-free localization
T. Hodson, J. Willsher, J. Knolle
Physical Review B 104 (4), 45116 (2021).
Disorder or interactions can turn metals into insulators. One of the simplest settings in which to study this physics is given by the Falikov-Kimball (FK) model, which describes itinerant fermions interacting with a classical Ising background field. Despite the translational invariance of the model, inhomogeneous configurations of the background field give rise to effective disorder physics which lead to a rich phase diagram in two (or more) dimensions with finite-temperature charge-density wave (CDW) transitions and interaction-tuned Anderson versus Mott localized phases. Here, we propose a generalized FK model in one dimension with long-range interactions which shows a similarly rich phase diagram. We use an exact Markov chain Monte Carlo method to map the phase diagram and compute the energy-resolved localization properties of the fermions. We compare the behavior of this transitionally invariant model to an Anderson model of uncorrelated binary disorder about a background CDW field which confirms that the fermionic sector only fully localizes for very large system sizes.
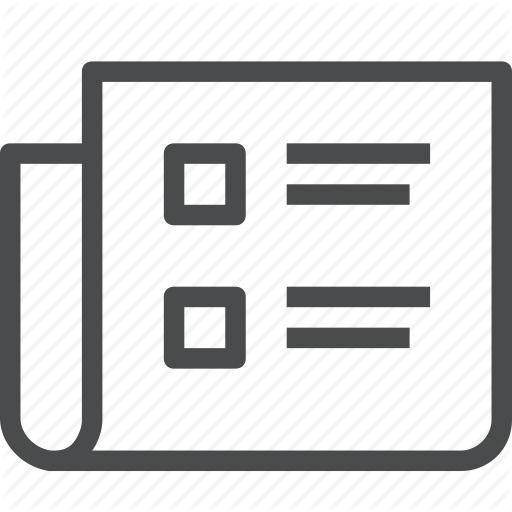
Flat and correlated plasmon bands in graphenek/alpha-RuCl3 heterostructures
H. K. Jin, J. Knolle
Physical Review B 104 (4), 45140 (2021).
We develop a microscopic theory for plasmon excitations of graphene/alpha-RuCl3 heterostructures. Within a Kondo-Kitaev model with various interactions, a heavy Fermi liquid hosting flat bands emerges in which the itinerant electrons of graphene effectively hybridize with the fractionalized fermions of the Kitaev quantum spin liquid. We find novel correlated plasmon bands induced by the interplay of flat bands and interactions and argue that our theory is consistent with the available experimental data on graphene/alpha-RuCl3 heterostructures. We predict novel plasmon branches beyond the long-wavelength limit and discuss the implications for probing correlation phenomena in other flat band systems.
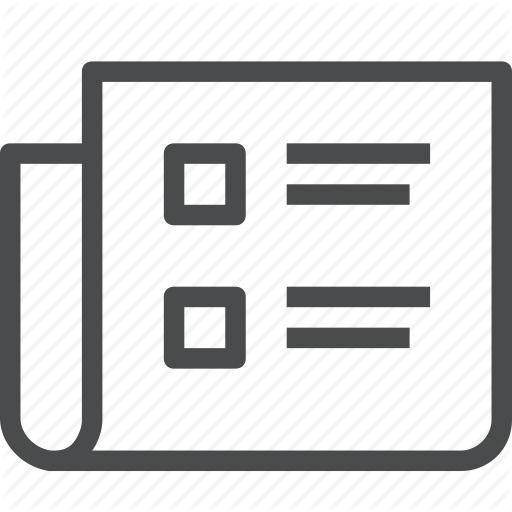
Confinement and entanglement dynamics on a digital quantum computer
J. Vovrosh, J. Knolle
Scientific Reports 11 (1), 11577 (2021).
Confinement describes the phenomenon when the attraction between two particles grows with their distance, most prominently found in quantum chromodynamics (QCD) between quarks. In condensed matter physics, confinement can appear in quantum spin chains, for example, in the one dimensional transverse field Ising model (TFIM) with an additional longitudinal field, famously observed in the quantum material cobalt niobate or in optical lattices. Here, we establish that state-of-the-art quantum computers have reached capabilities to simulate confinement physics in spin chains. We report quantitative confinement signatures of the TFIM on an IBM quantum computer observed via two distinct velocities for information propagation from domain walls and their mesonic bound states. We also find the confinement induced slow down of entanglement spreading by implementing randomized measurement protocols for the second order Renyi entanglement entropy. Our results are a crucial step for probing non-perturbative interacting quantum phenomena on digital quantum computers beyond the capabilities of classical hardware.
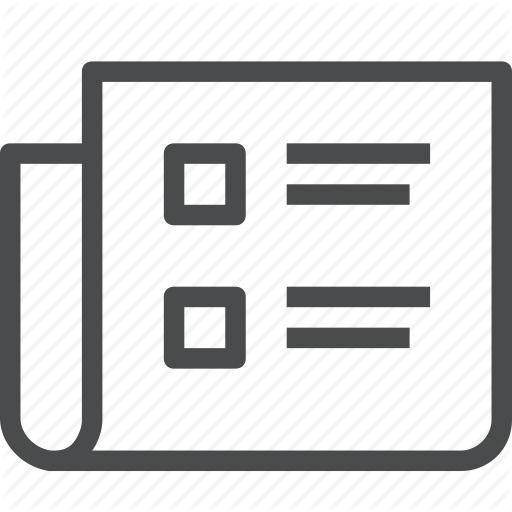
Higher-order and fractional discrete time crystals in clean long-range interacting systems
A. Pizzi, J. Knolle, A. Nunnenkamp
Nature Communications 12 (1), 2341 (2021).
Discrete time crystals are periodically driven systems characterized by a response with periodicity nT, with T the period of the drive and n>1. Typically, n is an integer and bounded from above by the dimension of the local (or single particle) Hilbert space, the most prominent example being spin-1/2 systems with n restricted to 2. Here, we show that a clean spin-1/2 system in the presence of long-range interactions and transverse field can sustain a huge variety of different 'higher-order' discrete time crystals with integer and, surprisingly, even fractional n>2. We characterize these (arguably prethermal) non-equilibrium phases of matter thoroughly using a combination of exact diagonalization, semiclassical methods, and spin-wave approximations, which enable us to establish their stability in the presence of competing long- and short-range interactions. Remarkably, these phases emerge in a model with continous driving and time-independent interactions, convenient for experimental implementations with ultracold atoms or trapped ions.
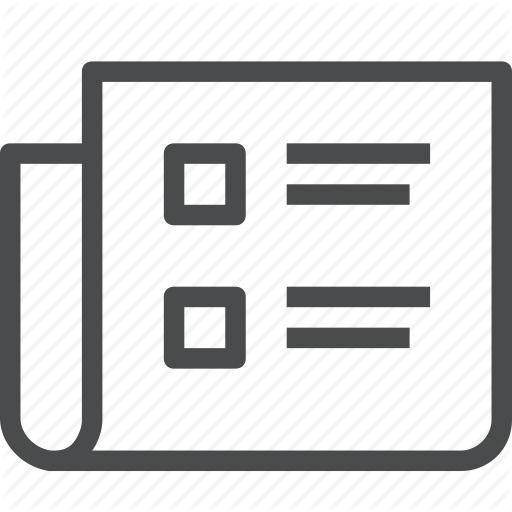
Gapless state of interacting Majorana fermions in a strain-induced Landau level
A. Agarwala, S. Bhattacharjee, J. Knolle, R. Moessner
Physical Review B 103 (13), 134427 (2021).
Mechanical strain can generate a pseudomagnetic field, and hence Landau levels (LL), for low-energy excitations of quantum matter in two dimensions. We study the collective state of the fractionalized Majorana fermions arising from residual generic spin interactions in the central LL, where the projected Hamiltonian reflects the spin symmetries in intricate ways: emergent U(1) and particle-hole symmetries forbid any bilinear couplings, leading to an intrinsically strongly interacting system,. also, they allow the definition of a filling fraction, which is fixed at 1/2. We argue that the resulting many-body state is gapless within our numerical accuracy, implying ultra-short-ranged spin correlations, while chirality correlators decay algebraically. This amounts to a Kitaev 'non-Fermi' spin liquid and shows that interacting Majorana Fermions can exhibit intricate behavior akin to fractional quantum Hall physics in an insulating magnet.
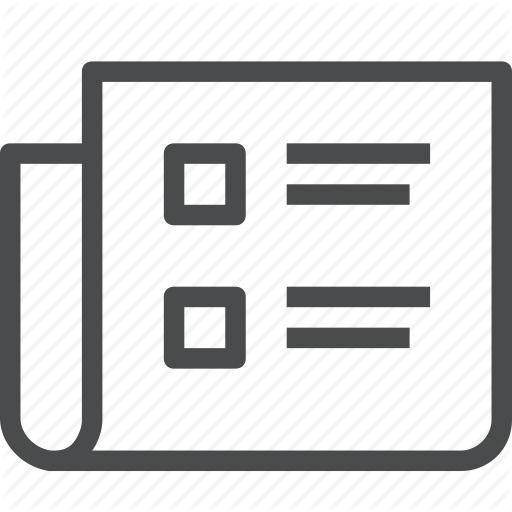
Butterfly effect and spatial structure of information spreading in a chaotic cellular automaton
S. W. Liu, J. Willsher, T. Bilitewski, J. J. Li, A. Smith, K. Christensen, R. Moessner, J. Knolle
Physical Review B 103 (9), 94109 (2021).
Inspired by recent developments in the study of chaos in many-body systems, we construct a measure of local information spreading for a stochastic cellular automaton in the form of a spatiotemporally resolved Hamming distance. This decorrelator is a classical version of an out-of-time-order correlator studied in the context of quantum many-body systems. Focusing on the one-dimensional Kauffman cellular automaton, we extract the scaling form of our decorrelator with an associated butterfly velocity vb and a velocity-dependent Lyapunov exponent lambda(v). The existence of the latter is not a given in a discrete classical system. Second, we account for the behavior of the decorrelator in a framework based solely on the boundary of the information spreading, including an effective boundary random walk model yielding the full functional form of the decorrelator. In particular, we obtain analytic results for v(b) and the exponent beta in the scaling ansatz lambda(v) similar to mu(v - v(b))(beta), which is usually only obtained numerically. Finally, a full scaling collapse establishes the decorrelator as a unifying diagnostic of information spreading.
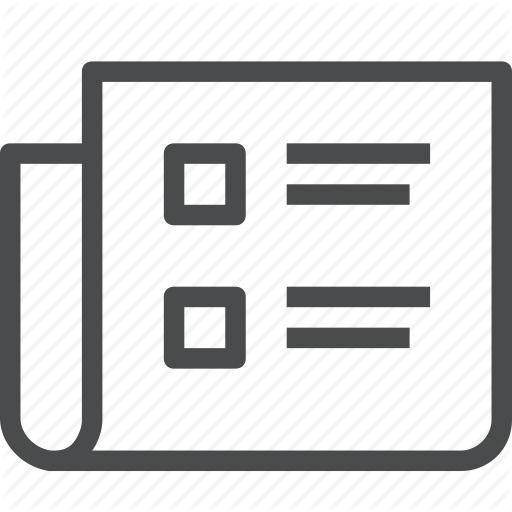
Anomalous Quantum Oscillations in a Heterostructure of Graphene on a Proximate Quantum Spin Liquid
V. Leeb, K. Polyudov, S. Mashhadi, S. Biswas, R. Valenti, M. Burghard, J. Knolle
Physical Review Letters 126 (9), 97201 (2021).
The quasi-two-dimensional Mott insulator alpha-RuCl3 is proximate to the sought-after Kitaev quantum spin liquid (QSL). In a layer of alpha-RuCl3 on graphene, the dominant Kitaev exchange is further enhanced by strain. Recently, quantum oscillation (QO) measurements of such alpha-RuCl3 and graphene heterostructures showed an anomalous temperature dependence beyond the standard Lifshitz-Kosevich (LK) description. Here, we develop a theory of anomalous QO in an effective Kitaev-Kondo lattice model in which the itinerant electrons of the graphene layer interact with the correlated magnetic layer via spin interactions. At low temperatures, a heavy Fermi liquid emerges such that the neutral Majorana fermion excitations of the Kitaev QSL acquire charge by hybridizing with the graphene Dirac band. Using ab initio calculations to determine the parameters of our low-energy model, we provide a microscopic theory of anomalous QOs with a non-LK temperature dependence consistent with our measurements. We show how remnants of fractionalized spin excitations can give rise to characteristic signatures in QO experiments.
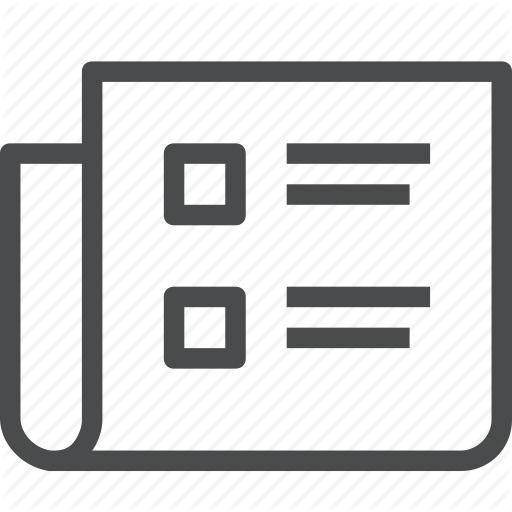
Bistability and time crystals in long-ranged directed percolation
A. Pizzi, A. Nunnenkamp, J. Knolle
Nature Communications 12 (1), 1061 (2021).
Stochastic processes govern the time evolution of a huge variety of realistic systems throughout the sciences. A minimal description of noisy many-particle systems within a Markovian picture and with a notion of spatial dimension is given by probabilistic cellular automata, which typically feature time-independent and short-ranged update rules. Here, we propose a simple cellular automaton with power-law interactions that gives rise to a bistable phase of long-ranged directed percolation whose long-time behaviour is not only dictated by the system dynamics, but also by the initial conditions. In the presence of a periodic modulation of the update rules, we find that the system responds with a period larger than that of the modulation for an exponentially (in system size) long time. This breaking of discrete time translation symmetry of the underlying dynamics is enabled by a self-correcting mechanism of the long-ranged interactions which compensates noise-induced imperfections. Our work thus provides a firm example of a classical discrete time crystal phase of matter and paves the way for the study of novel non-equilibrium phases in the unexplored field of driven probabilistic cellular automata. A model of a classical discrete time crystal satisfying the criteria of persistent subharmonic response robust against thermal noise and defects has been lacking. Here, the authors show that these criteria are satisfied in one-dimensional probabilistic cellular automata with long-range interactions and bistability.
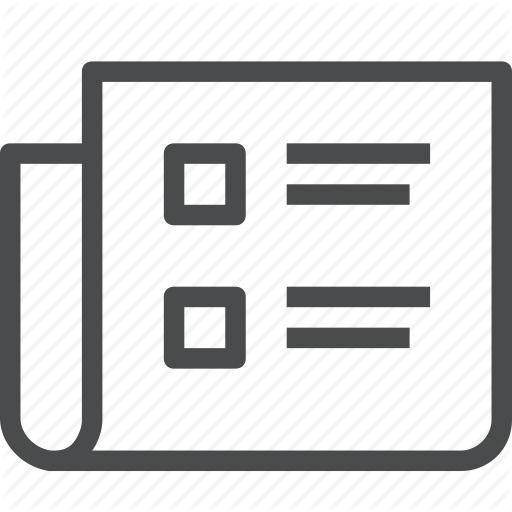
Seasonal epidemic spreading on small-world networks: Biennial outbreaks and classical discrete time crystals
D. Malz, A. Pizzi, A. Nunnenkamp, J. Knolle
Physical Review Research 3 (1), 13124 (2021).
We study seasonal epidemic spreading in a susceptible-infected-removed-susceptible model on small-world graphs. We derive a mean-field description that accurately captures the salient features of the model, most notably a phase transition between annual and biennial outbreaks. A numerical scaling analysis exhibits a diverging autocorrelation time in the thermodynamic limit, which confirms the presence of a classical discrete time crystalline phase. We derive the phase diagram of the model both frommean-field theory and from numerics. Our paper demonstrates that small worldness and non-Markovianity can stabilize a classical discrete time crystal, and links recent efforts to understand such dynamical phases of matter to the century-old problem of biennial epidemics.
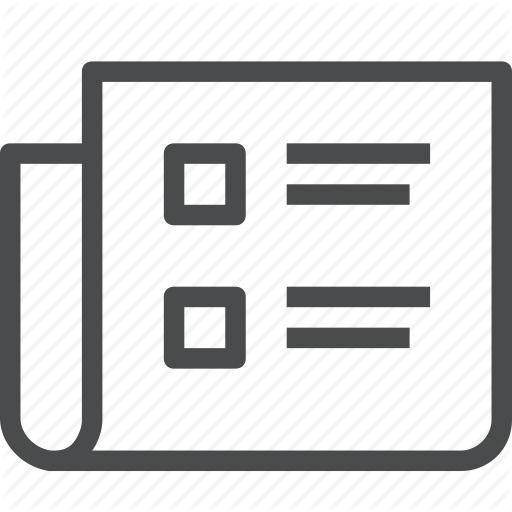
Vacancy-Induced Low-Energy Density of States in the Kitaev Spin Liquid
W. H. Kao, J. Knolle, G. B. Halasz, R. Moessner, N. B. Perkins
Physical Review X 11 (1), 11034 (2021).
The Kitaev honeycomb model has attracted significant attention due to its exactly solvable spin-liquid ground state with fractionalized Majorana excitations and its possible materialization in magnetic Mott insulators with strong spin-orbit couplings. Recently, the 5d-electron compound H3LiIr2O6 has shown to be a strong candidate for Kitaev physics considering the absence of any signs of a long-range ordered magnetic state. In this work, we demonstrate that a finite density of random vacancies in the Kitaev model gives rise to a striking pileup of low-energy Majorana eigenmodes and reproduces the apparent power-law upturn in the specific heat measurements of H3LiIr2O6. Physically, the vacancies can originate from various sources such as missing magnetic moments or the presence of nonmagnetic impurities (true vacancies), or from local weak couplings of magnetic moments due to strong but rare bond randomness (quasivacancies). We show numerically that the vacancy effect is readily detectable even at low vacancy concentrations and that it is not very sensitive either to the nature of vacancies or to different flux backgrounds. We also study the response of the site-diluted Kitaev spin liquid to the three-spin interaction term, which breaks time-reversal symmetry and imitates an external magnetic field. We propose a field-induced flux-sector transition where the ground state becomes flux-free for larger fields, resulting in a clear suppression of the low-temperature specific heat. Finally, we discuss the effect of dangling Majorana fermions in the case of true vacancies and show that their coupling to an applied magnetic field via the Zeeman interaction can also account for the scaling behavior in the high-field limit observed in H3LiIr2O6.
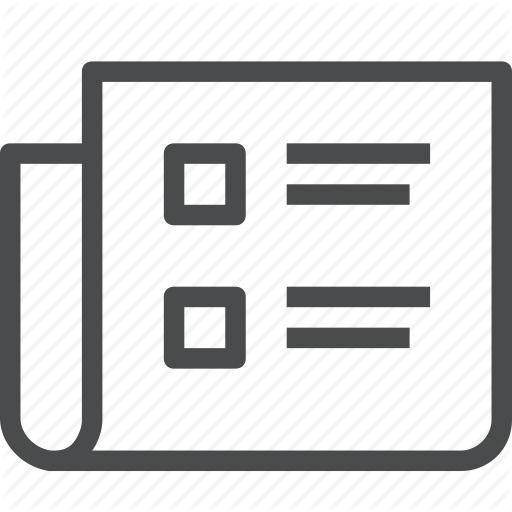
Random Multipolar Driving: Tunably Slow Heating through Spectral Engineering
H. Z. Zhao, F. Mintert, R. Moessner, J. Knolle
Physical Review Letters 126 (4), 40601 (2021).
Driven quantum systems may realize novel phenomena absent in static systems, but driving-induced heating can limit the timescale on which these persist. We study heating in interacting quantum many-body systems driven by random sequences with n-multipolar correlations, corresponding to a polynomially suppressed low-frequency spectrum. For n >= 1, we find a prethermal regime, the lifetime of which grows algebraically with the driving rate, with exponent 2n + 1. A simple theory based on Fermi's golden rule accounts for this behavior. The quasiperiodic Thue-Morse sequence corresponds to the n -> infinity limit and, accordingly, exhibits an exponentially long-lived prethermal regime. Despite the absence of periodicity in the drive, and in spite of its eventual heat death, the prethermal regime can host versatile nonequilibrium phases, which we illustrate with a random multipolar discrete time crystal.
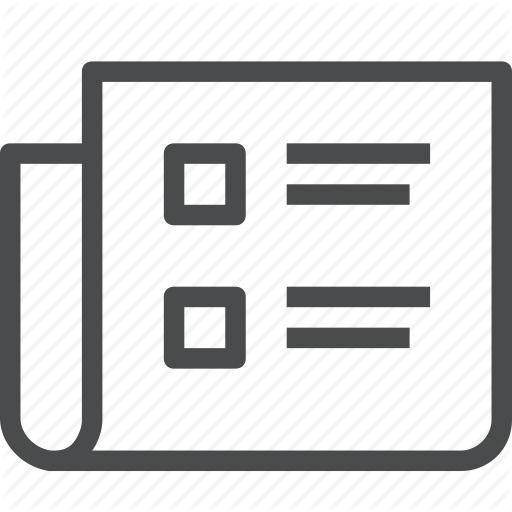
Time crystallinity and finite-size effects in clean Floquet systems
A. Pizzi, D. Malz, G. De Tomasi, J. Knolle, A. Nunnenkamp
Physical Review B 102 (21), 214207 (2020).
A cornerstone assumption that most literature on discrete time crystals has relied on is that homogeneous Floquet systems generally heat to a featureless infinite temperature state, an expectation that motivated researchers in the field to mostly focus on many-body localized systems. Some works have, however, shown that the standard diagnostics for time crystallinity apply equally well to clean settings without disorder. This fact raises the question whether a homogeneous discrete time crystal is possible in which the originally expected heating is evaded. Studying both a localized and an homogeneous model with short-range interactions, we clarify this issue showing explicitly the key differences between the two cases. On the one hand, our careful scaling analysis confirms that, in the thermodynamic limit and in contrast to localized discrete time crystals, homogeneous systems indeed heat. On the other hand, we show that, thanks to a mechanism reminiscent of quantum scars, finite-size homogeneous systems can still exhibit very crisp signatures of time crystallinity. A subharmonic response can in fact persist over timescales that are much larger than those set by the integrability-breaking terms, with thermalization possibly occurring only at very large system sizes (e.g., of hundreds of spins). Beyond clarifying the emergence of heating in disorder-free systems, our work casts a spotlight on finite-size homogeneous systems as prime candidates for the experimental implementation of nontrivial out-of-equilibrium physics.
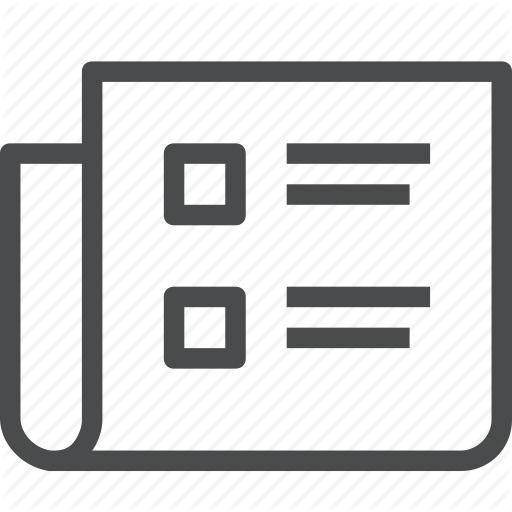
Magneto-optical conductivity in generic Weyl semimetals
M. Stalhammar, J. Larana-Aragon, J. Knolle, E. J. Bergholtz
Physical Review B 102 (23), 235134 (2020).
Magneto-optical studies of Weyl semimetals have been proposed as a versatile tool for observing low-energy Weyl fermions in candidate materials including the chiral Landau level. However, previous theoretical results have been restricted to the linearized regime around the Weyl node and are at odds with experimental findings. Here, we derive a closed form expression for the magneto-optical conductivity of generic Weyl semimetals in the presence of an external magnetic field aligned with the tilt of the spectrum. The systems are taken to have linear dispersion in two directions, while the tilting direction can consist of any arbitrary continuously differentiable function. This general calculation is then used to analytically evaluate the magneto-optical conductivity of Weyl semimetals expanded to cubic order in momentum. In particular, systems with arbitrary tilt, as well as systems hosting trivial Fermi pockets are investigated. The higher-order terms in momentum close the Fermi pockets in the type-II regime, removing the need for unphysical cutoffs when evaluating the magneto-optical conductivity. Crucially, the ability to take into account closed over-tilted and additional trivial Fermi pockets allows us to treat model systems closer to actual materials and we propose a simple explanation why the presence of parasitic trivial Fermi pockets can mask the characteristic signature of Weyl fermions in magneto-optical conductivity measurements.
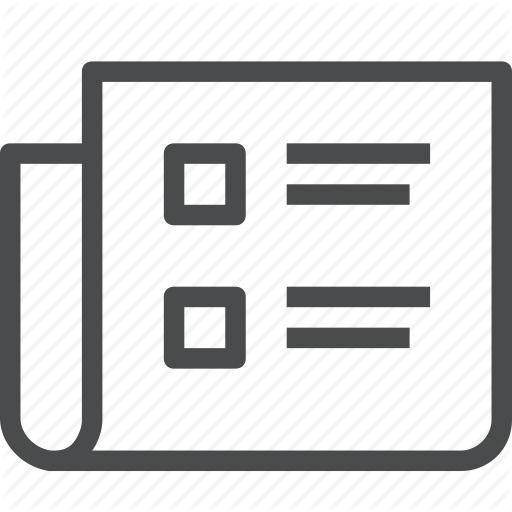
Disorder-free localization in a simple U (1) lattice gauge theory
I. Papaefstathiou, A. Smith, J. Knolle
Physical Review B 102 (16), 165132 (2020).
Localization due to the presence of disorder has proven crucial for our current understanding of relaxation in isolated quantum systems. The many-body localized phase constitutes a robust alternative to the thermalization of complex interacting systems, but recently the importance of disorder has been brought into question. A number of disorder-free localization mechanisms have been put forward connected to local symmetries of lattice gauge theories. Here, starting from translationally invariant (1 + 1)-dimensional quantum electrodynamics, we modify the dynamics of the gauge field which allows us to construct a lattice model with a U(1) local gauge symmetry revealing a mechanism of disorder-free localization. We consider two different discretizations of the continuum model resulting in a free-fermion soluble model in one case and an interacting model in the other. We diagnose the localization of our translationally invariant model in the far-from-equilibrium dynamics following a global quantum quench.
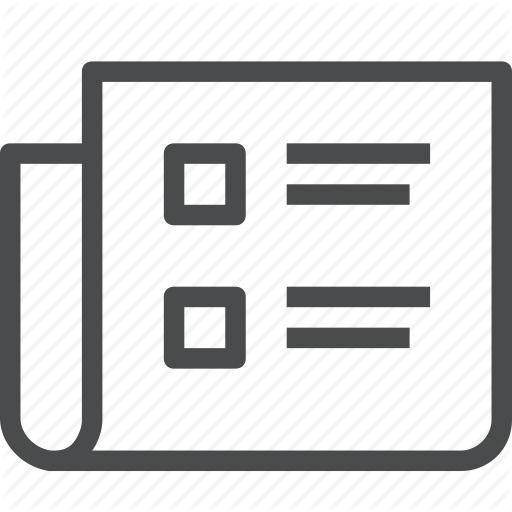
Local probes for charge-neutral edge states in two-dimensional quantum magnets
J. Feldmeier, W. Natori, M. Knap, J. Knolle
Physical Review B 102 (13), 134423 (2020).
The bulk-boundary correspondence is a defining feature of topological states of matter. However, for quantum magnets in two dimensions such as spin liquids or topological magnon insulators, a direct observation of topological surface states has proven challenging because of the charge-neutral character of the excitations. Here we propose spin-polarized scanning tunneling microscopy as a spin-sensitive local probe to provide direct information about charge-neutral topological edge states. We show how their signatures, imprinted in the local structure factor, can be extracted by specifically employing the strengths of existing technologies. As our main example, we determine the dynamical spin correlations of the Kitaev honeycomb model with open boundaries. We show that by contrasting conductance measurements of bulk and edge locations, one can extract direct signatures of the existence of fractionalized excitations and nontrivial topology. The broad applicability of this approach is corroborated by a second example of a kagome topological magnon insulator.
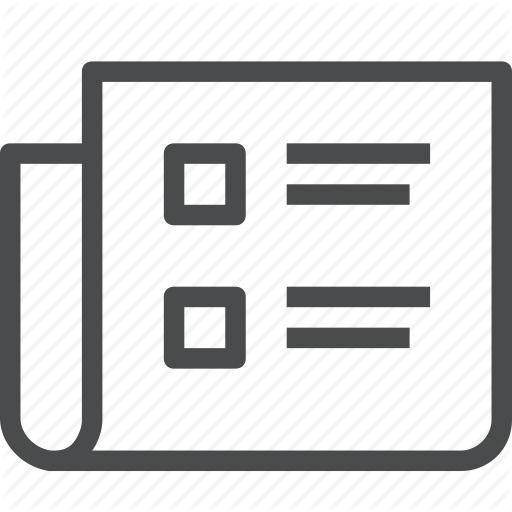
Dynamics of a Two-Dimensional Quantum Spin-Orbital Liquid: Spectroscopic Signatures of Fermionic Magnons
W. M. H. Natori, J. Knolle
Physical Review Letters 125 (6), 67201 (2020).
We provide an exact study of dynamical correlations for the quantum spin-orbital liquid phases of an SU(2)-symmetric Kitaev honeycomb lattice model. We show that the spin dynamics in this Kugel-Khomskii type model is exactly the density-density correlation function of S = 1 fermionic magnons, which could be probed in resonant inelastic x-ray scattering experiments. We predict the characteristic signatures of spin-orbital fractionalization in inelastic scattering experiments and compare them to the ones of the spin-anisotropic Kitaev honeycomb spin liquid. In particular, the resonant inelastic x-ray scattering response shows a characteristic momentum dependence directly related to the dispersion of fermionic excitations. The neutron scattering cross section displays a mixed response of fermionic magnons as well as spin-orbital excitations. The latter has a bandwidth of broad excitations and a vison gap that is three times larger than that of the spin-1 = 2 Kitaev model.
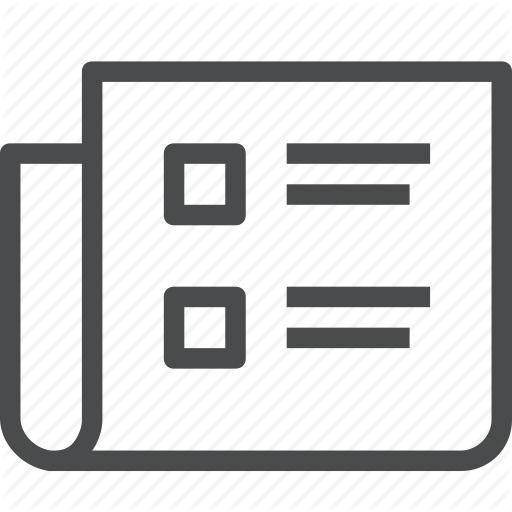
Quantum Many-Body Scars in Optical Lattices
H. Z. Zhao, J. Vovrosh, F. Mintert, J. Knolle
Physical Review Letters 124 (16), 160604 (2020).
The concept of quantum many-body scars has recently been put forward as a route to describe weak ergodicity breaking and violation of the eigenstate thermalization hypothesis. We propose a simple setup to generate quantum many-body scars in a doubly modulated Bose-Hubbard system which can be readily implemented in cold atomic gases. The dynamics are shown to be governed by kinetic constraints which appear via density-assisted tunneling in a high-frequency expansion. We find the optimal driving parameters for the kinetically constrained hopping which leads to small isolated subspaces of scared eigenstates. The experimental signatures and the transition to fully thermalizing behavior as a function of driving frequency are analyzed.
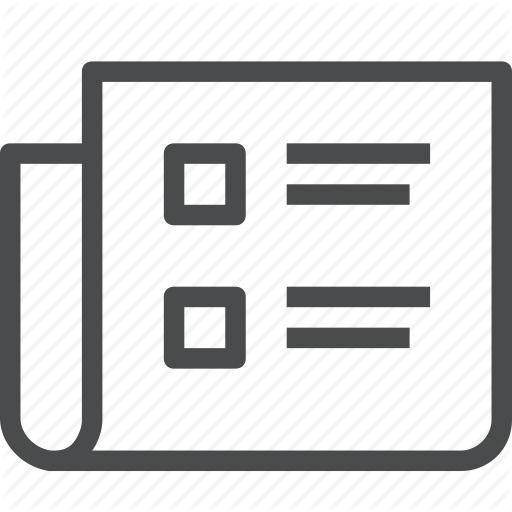
Electronic Properties of alpha-RuCl3 in Proximity to Graphene
S. Biswas, Y. Li, S. M. Winter, J. Knolle, R. Valenti
Physical Review Letters 123 (23), 237201 (2019).
In the pursuit of developing routes to enhance magnetic Kitaev interactions in alpha-RuCl3, as well as probing doping effects, we investigate the electronic properties of alpha-RuCl3 in proximity to graphene. We study alpha-RuCl3/graphene heterostructures via ab initio density functional theory calculations, Wannier projection, and nonperturbative exact diagonalization methods. We show that alpha-RuCl3 becomes strained when placed on graphene and charge transfer occurs between the two layers, making alpha-RuCl3 (graphene) lightly electron doped (hole doped). This gives rise to an insulator-to-metal transition in alpha-RuCl3 with the Fermi energy located close to the bottom of the upper Hubbard band of the t(2g) manifold. These results suggest the possibility of realizing metallic and even exotic superconducting states. Moreover, we show that in the strained alpha-RuCl3 monolayer the Kitaev interactions are enhanced by more than 50% compared to the unstrained bulk structure. Finally, we discuss scenarios related to transport experiments in alpha-RuCl3/graphene heterostructures.
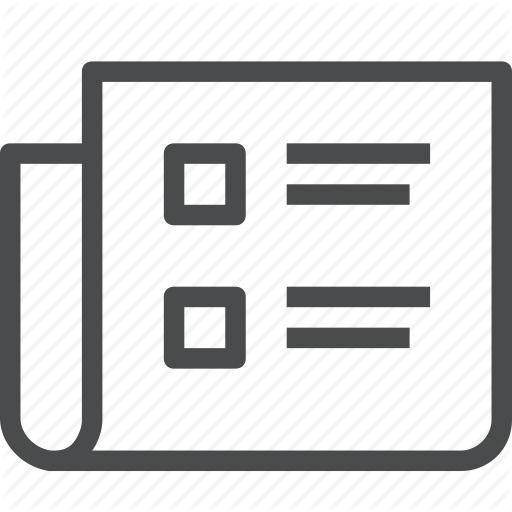
Period-n Discrete Time Crystals and Quasicrystals with Ultracold Bosons
A. Pizzi, J. Knolle, A. Nunnenkamp
Physical Review Letters 123 (15), 150601 (2019).
"We investigate the out-of-equilibrium properties of a system of interacting bosons in a ring lattice. We present a Floquet driving that induces clockwise (counterclockwise) circulation of the particles among the odd (even) sites of the ring which can be mapped to a fully connected model of clocks of two counterrotating species. The clocklike motion of the particles is at the core of a period-n discrete time crystal where L = 2n is the number of lattice sites. In the presence of a ""staircaselike"" on-site potential, we report the emergence of a second characteristic timescale in addition to the period n-tupling. This new timescale depends on the microscopic parameters of the Hamiltonian and is incommensurate with the Floquet period, underpinning a dynamical phase we call ""time quasicrystal."" The rich dynamical phase diagram also features a thermal phase and an oscillatory phase, all of which we investigate and characterize. Our simple, yet rich model can be realized with state-of-the-art ultracold atoms experiments."