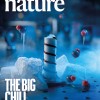
Evaporation of microwave-shielded polar molecules to quantum degeneracy
A. Schindewolf, R.Bause, X.-Y. Chen, M. Duda, T. Karman, I. Bloch & X.-Y. Luo
Nature 607, 677–681 (2022).
Ultracold polar molecules offer strong electric dipole moments and rich internal structure, which makes them ideal building blocks to explore exotic quantum matter1,2,3,4,5,6,7,8,9, implement quantum information schemes10,11,12 and test the fundamental symmetries of nature13. Realizing their full potential requires cooling interacting molecular gases deeply into the quantum-degenerate regime. However, the intrinsically unstable collisions between molecules at short range have so far prevented direct cooling through elastic collisions to quantum degeneracy in three dimensions. Here we demonstrate evaporative cooling of a three-dimensional gas of fermionic sodium–potassium molecules to well below the Fermi temperature using microwave shielding. The molecules are protected from reaching short range with a repulsive barrier engineered by coupling rotational states with a blue-detuned circularly polarized microwave. The microwave dressing induces strong tunable dipolar interactions between the molecules, leading to high elastic collision rates that can exceed the inelastic ones by at least a factor of 460. This large elastic-to-inelastic collision ratio allows us to cool the molecular gas to 21 nanokelvin, corresponding to 0.36 times the Fermi temperature. Such cold and dense samples of polar molecules open the path to the exploration of many-body phenomena with strong dipolar interactions.
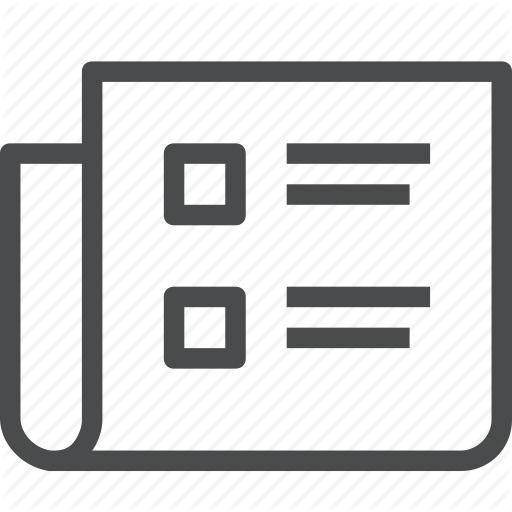
Practical quantum advantage in quantum simulation
A. J. Daley, I.Bloch, C. Kokail, S. Flannigan, N. Pearson, M.Troyer & P. Zoller .
Nature 607, 667–676 (2022).
The development of quantum computing across several technologies and platforms has reached the point of having an advantage over classical computers for an artificial problem, a point known as ‘quantum advantage’. As a next step along the development of this technology, it is now important to discuss ‘practical quantum advantage’, the point at which quantum devices will solve problems of practical interest that are not tractable for traditional supercomputers. Many of the most promising short-term applications of quantum computers fall under the umbrella of quantum simulation: modelling the quantum properties of microscopic particles that are directly relevant to modern materials science, high-energy physics and quantum chemistry. This would impact several important real-world applications, such as developing materials for batteries, industrial catalysis or nitrogen fixing. Much as aerodynamics can be studied either through simulations on a digital computer or in a wind tunnel, quantum simulation can be performed not only on future fault-tolerant digital quantum computers but also already today through special-purpose analogue quantum simulators. Here we overview the state of the art and future perspectives for quantum simulation, arguing that a first practical quantum advantage already exists in the case of specialized applications of analogue devices, and that fully digital devices open a full range of applications but require further development of fault-tolerant hardware. Hybrid digital–analogue devices that exist today already promise substantial flexibility in near-term applications.
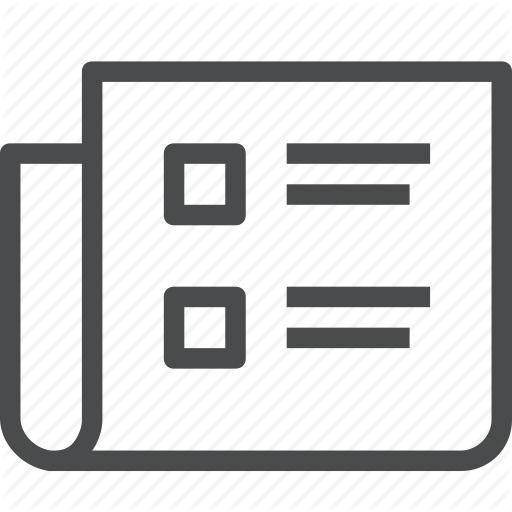
Benchmarking a Novel Efficient Numerical Method for Localized 1D Fermi-Hubbard Systems on a Quantum Simulator
B.H. Madhusudhana, S. Scherg, T. Kohlert, I. Bloch, M. Aidelsburger
Prx Quantum 2, 040325 (2021).
Quantum simulators have made a remarkable progress towards exploring the dynamics of many-body systems, many of which offer a formidable challenge to both theoretical and numerical methods. While state-of-the-art quantum simulators are, in principle, able to simulate quantum dynamics well outside the domain of classical computers, they are noisy and limited in the variability of the initial state of the dynamics and the observables that can be measured. Despite these limitations, here we show that such a quantum simulator can be used to in effect solve for the dynamics of a many-body system. We develop an efficient numerical technique that facilitates classical simulations in regimes not accessible to exact calculations or other established numerical techniques. The method is based on approximations that are well suited to describe localized one-dimensional Fermi-Hubbard systems. Since this new method does not have an error estimate and the approximations do not hold in general, we use a neutral-atom Fermi-Hubbard quantum simulator with
L
exp
≃
290
lattice sites to benchmark its performance in terms of accuracy and convergence for evolution times up to
700
tunneling times. We then use these approximations in order to derive a simple prediction of the behavior of interacting Bloch oscillations for spin-imbalanced Fermi-Hubbard systems, which we show to be in quantitative agreement with experimental results. Finally, we demonstrate that the convergence of our method is the slowest when the entanglement depth developed in the many-body system we consider is neither too small nor too large. This represents a promising regime for near-term applications of quantum simulators.
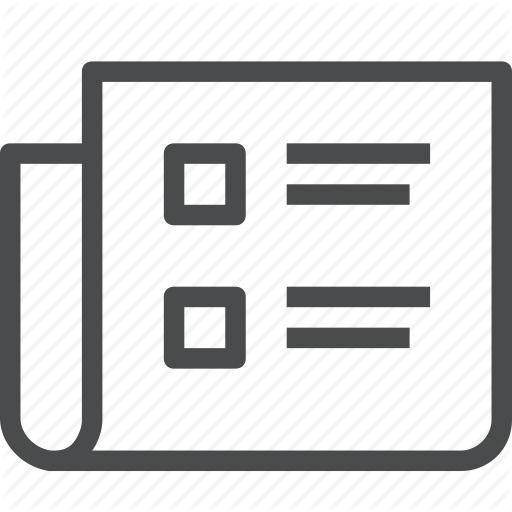
Efficient conversion of closed-channel-dominated Feshbach molecules of (NaK)-Na-23-K-40 to their absolute ground state
R. Bause, A. Kamijo, X.-Y. Chen, M. Duda, A. Schindewolf, I. Bloch, and X.-Y. Luo
Physical Review A 104, 043321 (2021).
We demonstrate the transfer of 23Na40K molecules from a closed-channel-dominated Feshbach-molecule state to the absolute ground state. The Feshbach molecules are initially created from a gas of sodium and potassium atoms via adiabatic ramping over a Feshbach resonance at 78.3 G. The molecules are then transferred to the absolute ground state using stimulated Raman adiabatic passage with an intermediate state in the spin-orbit-coupled complex ∣∣c3Σ+,v=35,J=1⟩∼|B1Π,v=12,J=1⟩. Our measurements show that the pump transition dipole moment linearly increases with the closed-channel fraction. Thus, the pump-beam intensity can be two orders of magnitude lower than is necessary with open-channel-dominated Feshbach molecules. We also demonstrate that the phase noise of the Raman lasers can be reduced by filter cavities, significantly improving the transfer efficiency.
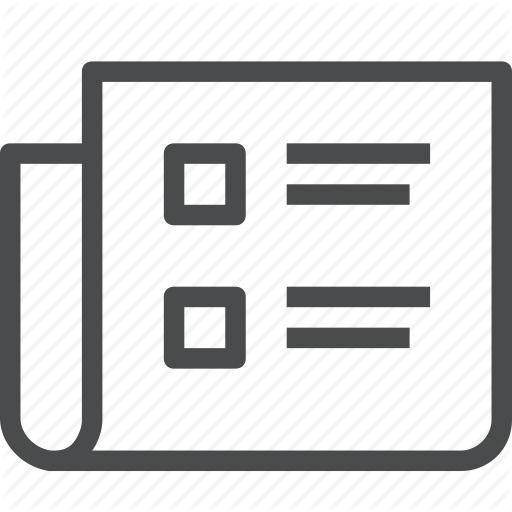
Microscopic evolution of doped Mott insulators from polaronic metal to Fermi liquid
J. Koepsell, D. Bourgund, P. Sompet, S. Hirthe, A. Bohrdt, Y. Wang, F. Grusdt, E. Demler, G. Salomon, C. Gross, I. Bloch
Science 374, 82-86 (2021).
The competition between antiferromagnetism and hole motion in two-dimensional Mott insulators lies at the heart of a doping-dependent transition from an anomalous metal to a conventional Fermi liquid. We observe such a crossover in Fermi-Hubbard systems on a cold-atom quantum simulator and reveal the transformation of multipoint correlations between spins and holes upon increasing doping at temperatures around the superexchange energy. Conventional observables, such as spin susceptibility, are furthermore computed from the microscopic snapshots of the system. Starting from a magnetic polaron regime, we find the system evolves into a Fermi liquid featuring incommensurate magnetic fluctuations and fundamentally altered correlations. The crossover is completed for hole dopings around 30%. Our work benchmarks theoretical approaches and discusses possible connections to lowertemperature phenomena.
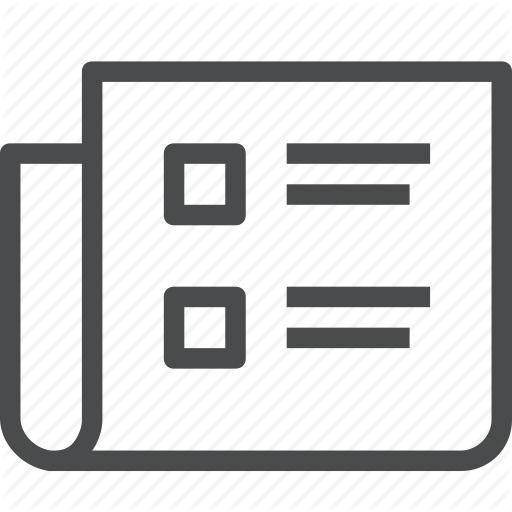
Observing non-ergodicity due to kinetic constraints in tilted Fermi-Hubbard chains
S. Scherg, T. Kohlert, P. Sala, F. Pollmann, H.M. Bharath, I. Bloch, M. Aidelsburger
Nature Communications 12, 4490 (2021).
The thermalization of isolated quantum many-body systems is deeply related to fundamental questions of quantum information theory. While integrable or many-body localized systems display non-ergodic behavior due to extensively many conserved quantities, recent theoretical studies have identified a rich variety of more exotic phenomena in between these two extreme limits. The tilted one-dimensional Fermi-Hubbard model, which is readily accessible in experiments with ultracold atoms, emerged as an intriguing playground to study non-ergodic behavior in a clean disorder-free system. While non-ergodic behavior was established theoretically in certain limiting cases, there is no complete understanding of the complex thermalization properties of this model. In this work, we experimentally study the relaxation of an initial charge-density wave and find a remarkably long-lived initial-state memory over a wide range of parameters. Our observations are well reproduced by numerical simulations of a clean system. Using analytical calculations we further provide a detailed microscopic understanding of this behavior, which can be attributed to emergent kinetic constraints.
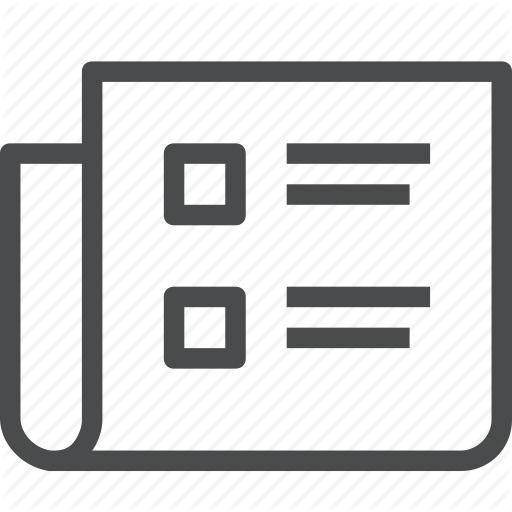
Quantum Information Processing and Precision Measurement Using a Levitated Nanodiamond
H.J. Zhang, X.Y. Chen, Z.Q. Yin
Advanced Quantum Technologies 4 (8), (2021).
The nanodiamond, which hosts nitrogen-vacancy (NV) centers, has been levitated in vacuum either through optical tweezers or through an ion trap. It combines the advantages of the large mass and long coherent discrete internal energy levels, which makes it an ideal platform for quantum information processing and precision measurement. In this review, the quantum information processing and the precision measurement based on the levitated nanodiamond are briefly summarized. The basic physics of the levitated nanodiamond and the NV centers is first introduced. Then the methods of manipulating motional states of the nanodiamond with the NV centers are discussed. The magnetic coupling mechanisms between the NV centers and the translational mode or the torsional mode are discussed, and the method of cooling the mechanical modes with the NV centers is discussed. Several applications are discussed, such as the mass spectrometry, the gravitational acceleration measurement, etc. The levitated nanodiamond can also be used for realizing universal quantum logic gates and quantum simulation. Finally, the conclusion and perspective are given.
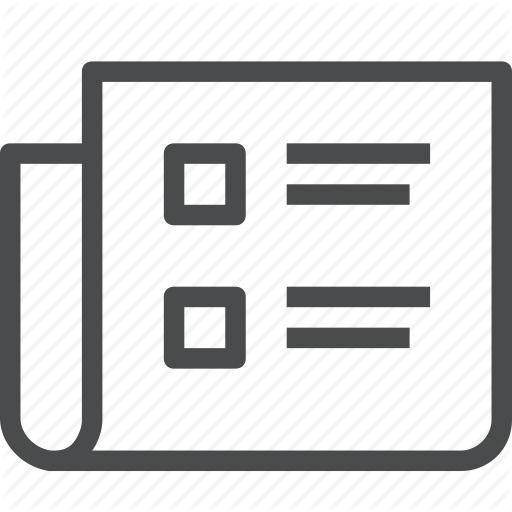
Collisions of ultracold molecules in bright and dark optical dipole traps
R. Bause, A. Schindewolf, R. Tao, M. Duda, X.-Y. Chen, G. Quéméner, T. Karman, A. Christianen, I. Bloch, X.-Y. Luo
Physical Review Research 3, 33013 (2021).
Understanding collisions between ultracold molecules is crucial for making stable molecular quantum gases and harnessing their rich internal degrees of freedom for quantum engineering. Transient complexes can strongly influence collisional physics, but in the ultracold regime, key aspects of their behavior have remained unknown. To explain experimentally observed loss of ground-state molecules from optical dipole traps, it was recently proposed that molecular complexes can be lost due to photo-excitation. By trapping molecules in a repulsive box potential using laser light near a narrow molecular transition, we are able to test this hypothesis with light intensities three orders of magnitude lower than what is typical in red-detuned dipole traps. This allows us to investigate light-induced collisional loss in a gas of nonreactive fermionic 23Na40K molecules. Even for the lowest intensities available in our experiment, our results are consistent with universal loss, meaning unit loss probability inside the short-range interaction potential. Our findings disagree by at least two orders of magnitude with latest theoretical predictions, showing that crucial aspects of molecular collisions are not yet understood, and provide a benchmark for the development of new theories.
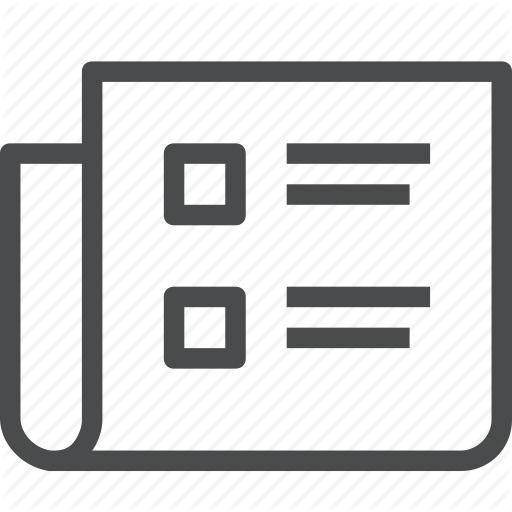
Microscopic electronic structure tomography of Rydberg macrodimers
S. Hollerith, J. Rui, A. Rubio-Abadal, K. Srakaew, D. Wei, J. Zeiher, C. Gross, I. Bloch
Rhys. Rev. Research 3, 13252 (2021).
Precise control and study of molecules is challenging due to the variety of internal degrees of freedom and local coordinates that are typically not controlled in an experiment. Employing quantum gas microscopy to position and resolve the atoms in Rydberg macrodimer states solves almost all of these challenges and enables unique access to the molecular frame. Here, we demonstrate the power of this approach and present first photoassociation studies for different molecular symmetries in which the molecular orientation relative to an applied magnetic field, the polarization of the excitation light and the initial atomic state are fully controlled. The observed characteristic dependencies allow for an electronic structure tomography of the molecular state. We additionally observe an orientation-dependent Zeeman shift and reveal a significant influence on it caused by the hyperfine interaction of the macrodimer state. Finally, we demonstrate controlled engineering of the electrostatic binding potential by opening a gap in the energetic vicinity of two crossing pair potentials.
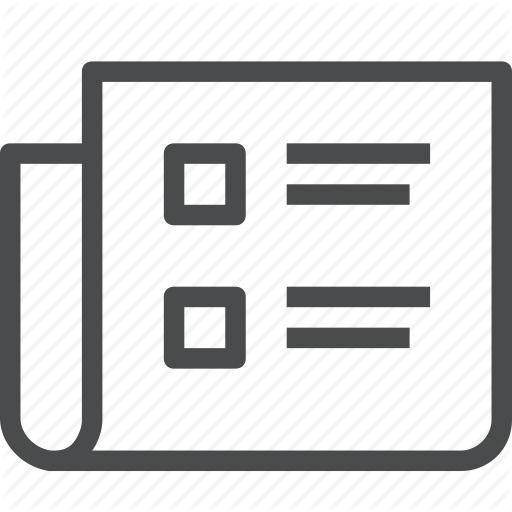
Crossed optical cavities with large mode diameters
A. Heinz, J. Trautmann, N. Šantić, A. J. Park, I. Bloch, S. Blatt
Optics Letters 46 (2), 250-253 (2021).
We report on a compact, ultrahigh-vacuum compatible optical assembly to create large-scale, two-dimensional optical lattices for use in experiments with ultracold atoms. The assembly consists of an octagon-shaped spacer made from ultra-low-expansion glass, to which we optically contact four fused-silica cavity mirrors, making it highly mechanically and thermally stable. The mirror surfaces are nearly plane-parallel which allows us to create two perpendicular cavity modes with diameters ∼1 mm. Such large mode diameters are desirable to increase the optical lattice homogeneity, but lead to strong angular sensitivities of the coplanarity between the two cavity modes. We demonstrate a procedure to precisely position each mirror substrate that achieves a deviation from coplanarity of d=1(5) μm. Creating large optical lattices at arbitrary visible and near infrared wavelengths requires significant power enhancements to overcome limitations in the available laser power. The cavity mirrors have a customized low-loss mirror coating that enhances the power at a set of relevant wavelengths from the visible to the near infrared by up to three orders of magnitude.
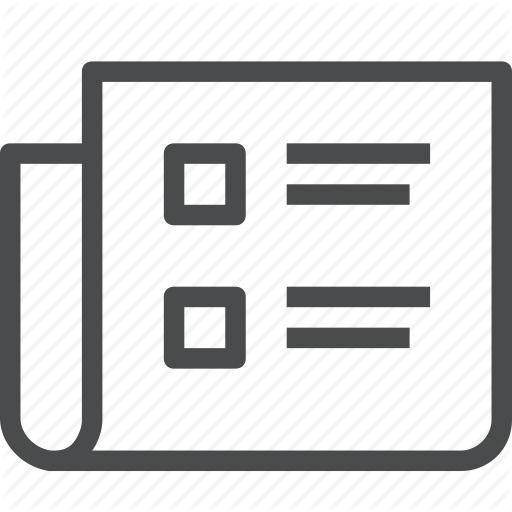
Quasiparticle Lifetime of the Repulsive Fermi Polaron
H.S. Adlong, W.E. Liu, F. Scazza, M. Zaccanti, N.D. Oppong, S. Foelling, M.M. Parish, J. Levinsen
Physical Review Letters 125 (13), 133401 (2020).
We investigate the metastable repulsive branch of a mobile impurity coupled to a degenerate Fermi gas via short-range interactions. We show that the quasiparticle lifetime of this repulsive Fermi polaron can be experimentally probed by driving Rabi oscillations between weakly and strongly interacting impurity states. Using a time-dependent variational approach, we find that we can accurately model the impurity Rabi oscillations that were recently measured for repulsive Fermi polarons in both two and three dimensions. Crucially, our theoretical description does not include relaxation processes to the lower-lying attractive branch. Thus, the theory-experiment agreement demonstrates that the quasiparticle lifetime is dominated by many-body dephasing within the upper repulsive branch rather than by relaxation from the upper branch itself. Our findings shed light on recent experimental observations of persistent repulsive correlations, and have important consequences for the nature and stability of the strongly repulsive Fermi gas.
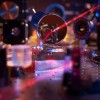
A subradiant optical mirror formed by a single structured atomic layer
J. Rui, D.V. Wei, A. Rubio-Abadal, S. Hollerith, J. Zeiher, D.M. Stamper-Kurn, C. Gross, I. Bloch
Nature 583 (7816), 369–374 (2020).
Versatile interfaces with strong and tunable light-matter interactions are essential for quantum science(1)because they enable mapping of quantum properties between light and matter(1). Recent studies(2-10)have proposed a method of controlling light-matter interactions using the rich interplay of photon-mediated dipole-dipole interactions in structured subwavelength arrays of quantum emitters. However, a key aspect of this approach-the cooperative enhancement of the light-matter coupling strength and the directional mirror reflection of the incoming light using an array of quantum emitters-has not yet been experimentally demonstrated. Here we report the direct observation of the cooperative subradiant response of a two-dimensional square array of atoms in an optical lattice. We observe a spectral narrowing of the collective atomic response well below the quantum-limited decay of individual atoms into free space. Through spatially resolved spectroscopic measurements, we show that the array acts as an efficient mirror formed by a single monolayer of a few hundred atoms. By tuning the atom density in the array and changing the ordering of the particles, we are able to control the cooperative response of the array and elucidate the effect of the interplay of spatial order and dipolar interactions on the collective properties of the ensemble. Bloch oscillations of the atoms outside the array enable us to dynamically control the reflectivity of the atomic mirror. Our work demonstrates efficient optical metamaterial engineering based on structured ensembles of atoms(4,8,9)and paves the way towards controlling many-body physics with light(5,6,11)and light-matter interfaces at the single-quantum level(7,10).
A single two-dimensional array of atoms trapped in an optical lattice shows a tunable cooperative subradiant optical response, acting as a single-monolayer optical mirror with controllable reflectivity.
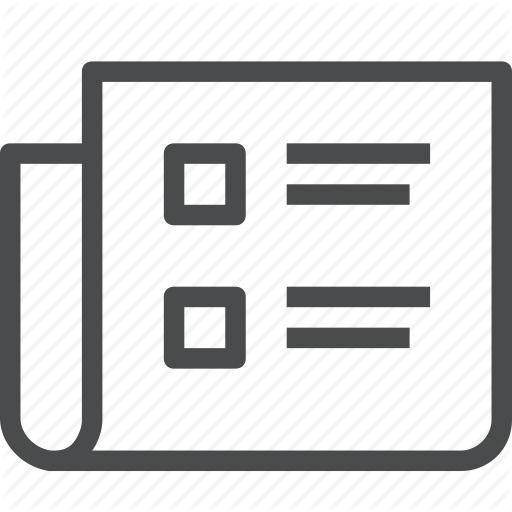
Tune-out and magic wavelengths for ground-state 23Na40K molecules
R. Bause, M. Li, A. Schindewolf, X.-Y. Chen, M. Duda, S. Kotochigova, I. Bloch, X.-Y. Luo
Physical Review Letters 125, 23201 (2020).
We demonstrate a versatile, state-dependent trapping scheme for the ground and first excited rotational states of 23Na40K molecules. Close to the rotational manifold of a narrow electronic transition, we determine tune-out frequencies where the polarizability of one state vanishes while the other remains finite, and a magic frequency where both states experience equal polarizability. The proximity of these frequencies of only 10 GHz allows for dynamic switching between different trap configurations in a single experiment, while still maintaining sufficiently low scattering rates.
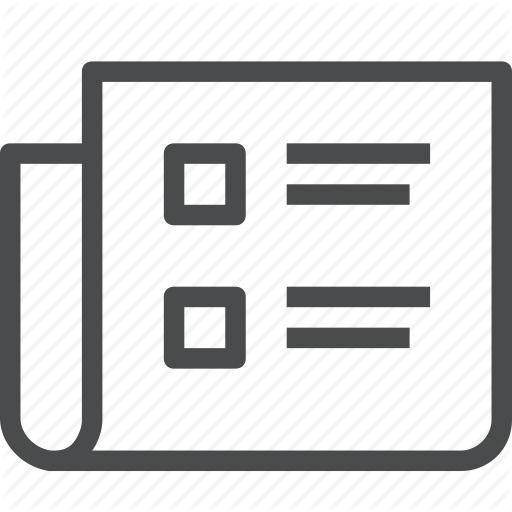
Robust Bilayer Charge Pumping for Spin- and Density-Resolved Quantum Gas Microscopy
J. Koepsell, S. Hirthe, D. Bourgund, P. Sompet, J. Vijayan, G. Salomon, C. Gross, I. Bloch
Physical Review Letters 125 (1), 010403 (2020).
Quantum gas microscopy has emerged as a powerful new way to probe quantum many-body systems at the microscopic level. However, layered or efficient spin-resolved readout methods have remained scarce as they impose strong demands on the specific atomic species and constrain the simulated lattice geometry and size. Here we present a novel high-fidelity bilayer readout, which can be used for full spin- and density-resolved quantum gas microscopy of two-dimensional systems with arbitrary geometry. Our technique makes use of an initial Stern-Gerlach splitting into adjacent layers of a highly stable vertical superlattice and subsequent charge pumping to separate the layers by 21 mu m. This separation enables independent high-resolution images of each layer. We benchmark our method by spin- and density-resolving two-dimensional Fermi-Hubbard systems. Our technique furthermore enables the access to advanced entropy engineering schemes, spectroscopic methods, or the realization of tunable bilayer systems.
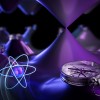
Realization of an anomalous Floquet topological system with ultracold atoms
K. Wintersperger, C. Braun, F. Nur Ünal, A. Eckardt, M. Di Liberto, N. Goldman, I. Bloch, M. Aidelsburger
Nature Physics (2020).
Coherent control via periodic modulation, also known as Floquet engineering, has emerged as a powerful experimental method for the realization of novel quantum systems with exotic properties. In particular, it has been employed to study topological phenomena in a variety of different platforms. In driven systems, the topological properties of the quasienergy bands can often be determined by standard topological invariants, such as Chern numbers, which are commonly used in static systems. However, due to the periodic nature of the quasienergy spectrum, this topological description is incomplete and new invariants are required to fully capture the topological properties of these driven settings. Most prominently, there are two-dimensional anomalous Floquet systems that exhibit robust chiral edge modes, despite all Chern numbers being equal to zero. Here we realize such a system with bosonic atoms in a periodically driven honeycomb lattice and infer the complete set of topological invariants from energy gap measurements and local Hall deflections.
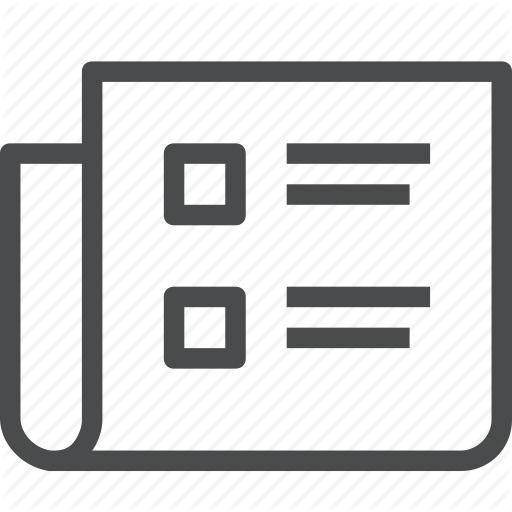
Floquet Prethermalization in a Bose-Hubbard System
A. Rubio-Abadal, M. Ippoliti, S. Hollerith, D. Wei, J, Rui, S.L. Sondhi, V. Khemani, C. Gross, I. Bloch
Physical Review X 10 (2), 021044 (2020).
Periodic driving has emerged as a powerful tool in the quest to engineer new and exotic quantum phases. While driven many-body systems are generically expected to absorb energy indefinitely and reach an infinite-temperature state, the rate of heating can be exponentially suppressed when the drive frequency is large compared to the local energy scales of the system-leading to long-lived "prethermal" regimes. In this work, we experimentally study a bosonic cloud of ultracold atoms in a driven optical lattice and identify such a prethermal regime in the Bose-Hubbard model. By measuring the energy absorption of the cloud as the driving frequency is increased, we observe an exponential-in-frequency reduction of the heating rate persisting over more than 2 orders of magnitude. The tunability of the lattice potentials allows us to explore one- and two-dimensional systems in a range of different interacting regimes. Alongside the exponential decrease, the dependence of the heating rate on the frequency displays features characteristic of the phase diagram of the Bose-Hubbard model, whose understanding is additionally supported by numerical simulations in one dimension. Our results show experimental evidence of the phenomenon of Floquet prethermalization and provide insight into the characterization of heating for driven bosonic systems.
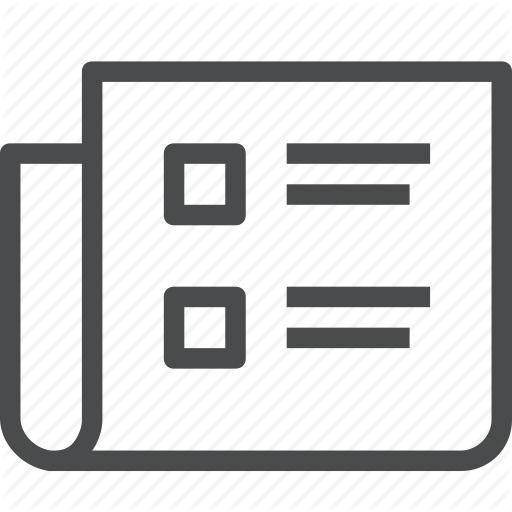
Orbital ordering of ultracold alkaline-earth atoms in optical lattices
A. Sotnikov, N.D. Oppong, Y. Zambrano, A. Cichy
Physical Review Research 2, 023188 (2020).
We report on a dynamical mean-field theoretical analysis of emerging low-temperature phases in multicomponent gases of fermionic alkaline-earth(-like) atoms in state-dependent optical lattices. Using the example of Yb-173 atoms, we show that a two-orbital mixture with two nuclear spin components is a promising candidate for studies of not only magnetic but also staggered orbital ordering peculiar to certain solid-state materials. We calculate and study the phase diagram of the full Hamiltonian with parameters similar to existing experiments and reveal an antiferro-orbital phase. This long-range-ordered phase is inherently stable, and we analyze the change of local and global observables across the corresponding transition lines, paving the way for experimental observations. Furthermore, we suggest a realistic extension of the system to include and probe a Jahn-Teller source field playing one of the key roles in real crystals.
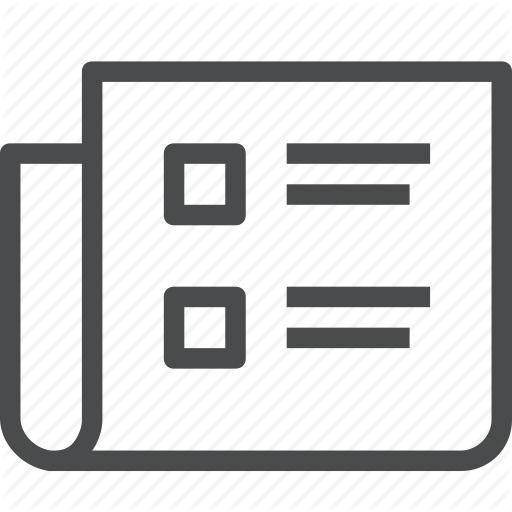
State-Dependent Optical Lattices for the Strontium Optical Qubit
A. Heinz, A. J. Park, N. Šantić, J. Trautmann, S. G. Porsev, M. S. Safronova, I. Bloch, S. Blatt
Physical Review Letters 124, 203201 (2020).
We demonstrate state-dependent optical lattices for the Sr optical qubit at the tune-out wavelength for its ground state. We tightly trap excited state atoms while suppressing the effect of the lattice on ground state atoms by more than four orders of magnitude. This highly independent control over the qubit states removes inelastic excited state collisions as the main obstacle for quantum simulation and computation schemes based on the Sr optical qubit. Our results also reveal large discrepancies in the atomic data used to calibrate the largest systematic effect of Sr optical lattice clocks.
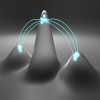
Parametric Instabilities of Interacting Bosons in Periodically Driven 1D Optical Lattices
K. Wintersperger, M. Bukov, J. Näger, S. Lellouch, E. Demler, U. Schneider, I. Bloch, N. Goldman, M. Aidelsburger
Physical Review X 10, 011030 (2020).
Periodically driven quantum systems are currently explored in view of realizing novel many-body phases of matter. This approach is particularly promising in gases of ultracold atoms, where sophisticated shaking protocols can be realized and interparticle interactions are well controlled. The combination of interactions and time-periodic driving, however, often leads to uncontrollable heating and instabilities, potentially preventing practical applications of Floquet engineering in large many-body quantum systems. In this work, we experimentally identify the existence of parametric instabilities in weakly interacting Bose-Einstein condensates in strongly driven optical lattices through momentum-resolved measurements, in line with theoretical predictions. Parametric instabilities can trigger the destruction of weakly interacting Bose-Einstein condensates through the rapid growth of collective excitations, in particular in systems with weak harmonic confinement transverse to the lattice axis. Understanding the onset of parametric instabilities in driven quantum matter is crucial for determining optimal conditions for the engineering of modulation-induced many-body systems.
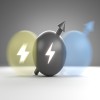
Time-resolved observation of spin-charge deconfinement in fermionic Hubbard chains
J. Vijayan, P. Sompet, G. Salomon, J. Koepsell, S. Hirthe, A. Bohrdt, F. Grusdt, I. Bloch, C. Gross
Science 10, 186-189 (2020).
Elementary particles carry several quantum numbers, such as charge and spin. However, in an ensemble of strongly interacting particles, the emerging degrees of freedom can fundamentally differ from those of the individual constituents. For example, one-dimensional systems are described by independent quasiparticles carrying either spin (spinon) or charge (holon). Here, we report on the dynamical deconfinement of spin and charge excitations in real space after the removal of a particle in Fermi-Hubbard chains of ultracold atoms. Using space- and time-resolved quantum gas microscopy, we tracked the evolution of the excitations through their signatures in spin and charge correlations. By evaluating multipoint correlators, we quantified the spatial separation of the excitations in the context of fractionalization into single spinons and holons at finite temperatures.
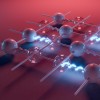
Floquet approach to Z2 lattice gauge theories with ultracold atoms in optical lattices
C. Schweizer, F. Grusdt, M. Berngruber, L. Barbiero, E. Demler, N. Goldman, I. Bloch, M. Aidelsburger.
Nature Physics 15, 1168-1173 (2019).
Quantum simulation has the potential to investigate gauge theories in strongly-interacting regimes, which are up to now inaccessible through conventional numerical techniques. Here, we take a first step in this direction by implementing a Floquet-based method for studying Z2 lattice gauge theories using two-component ultracold atoms in a double-well potential. For resonant periodic driving at the on-site interaction strength and an appropriate choice of the modulation parameters, the effective Floquet Hamiltonian exhibits Z2 symmetry. We study the dynamics of the system for different initial states and critically contrast the observed evolution with a theoretical analysis of the full time-dependent Hamiltonian of the periodically-driven lattice model. We reveal challenges that arise due to symmetry-breaking terms and outline potential pathways to overcome these limitations. Our results provide important insights for future studies of lattice gauge theories based on Floquet techniques.
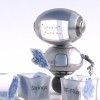
Classifying snapshots of the doped Hubbard model with machine learning
A. Bohrdt, C. S. Chiu, G. Ji, M. Xu, D. Greif, M. Greiner, E. Demler, F. Grusdt, M. Knap.
Nature Physics 15, 921-924 (2019).
Quantum gas microscopes for ultracold atoms can provide high-resolution real-space snapshots of complex many-body systems. We implement machine learning to analyse and classify such snapshots of ultracold atoms. Specifically, we compare the data from an experimental realization of the two-dimensional Fermi–Hubbard model to two theoretical approaches: a doped quantum spin liquid state of resonating valence bond type (1,2), and the geometric string theory (3,4), describing a state with hidden spin order. This technique considers all available information without a potential bias towards one particular theory by the choice of an observable and can therefore select the theory that is more predictive in general. Up to intermediate doping values, our algorithm tends to classify experimental snapshots as geometric-string-like, as compared to the doped spin liquid. Our results demonstrate the potential for machine learning in processing the wealth of data obtained through quantum gas microscopy for new physical insights.
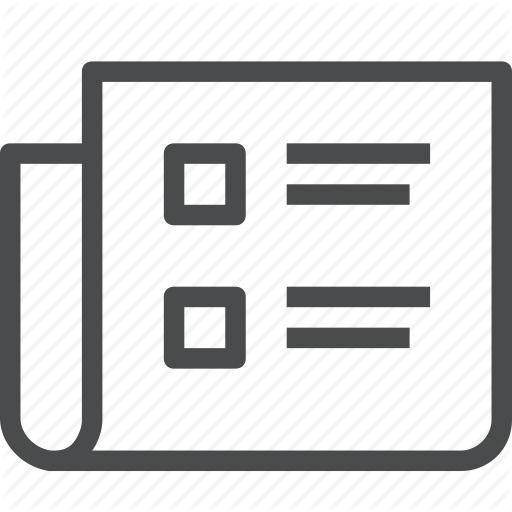
Quantum gas microscopy of Rydberg macrodimers
S. Hollerith, J. Zeiher, J. Rui, A. Rubio-Abadal, V. Walther, T. Pohl, D.M. Stamper-Kurn, I. Bloch, C. Gross
Science 364, 664-667 (2019).
A microscopic understanding of molecules is essential for many fields of natural sciences but their tiny size hinders direct optical access to their constituents. Rydberg macrodimers - bound states of two highly-excited Rydberg atoms - feature bond lengths easily exceeding optical wavelengths. Here we report on the direct microscopic observation and detailed characterization of such macrodimers in a gas of ultracold atoms in an optical lattice. The size of about 0.7 micrometers, comparable to the size of small bacteria, matches the diagonal distance of the lattice. By exciting pairs in the initial two-dimensional atom array, we resolve more than 50 vibrational resonances. Using our spatially resolved detection, we observe the macrodimers by correlated atom loss and demonstrate control of the molecular alignment by the choice of the vibrational state. Our results allow for precision testing of Rydberg interaction potentials and establish quantum gas microscopy as a powerful new tool for quantum chemistry.
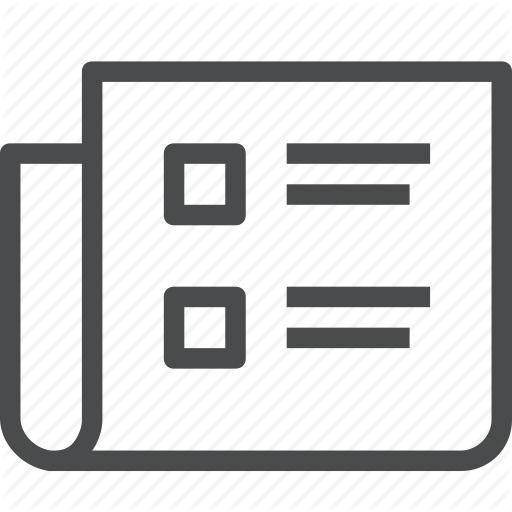
Observation of many-body localization in an one-dimensional system with a single-particle mobility edge
T. Kohlert, S. Scherg, X. Li, H.P. Lüschen, S. Das Sarma, I. Bloch, M. Aidelsburger.
Physical Review Letters 122, 170403 (2019).
We experimentally study many-body localization (MBL) with ultracold atoms in a weak one-dimensional quasiperiodic potential, which in the noninteracting limit exhibits an intermediate phase that is characterized by a mobility edge. We measure the time evolution of an initial charge density wave after a quench and analyze the corresponding relaxation exponents. We find clear signatures of MBL when the corresponding noninteracting model is deep in the localized phase. We also critically compare and contrast our results with those from a tight-binding Aubry-André model, which does not exhibit a single-particle intermediate phase, in order to identify signatures of a potential many-body intermediate phase.