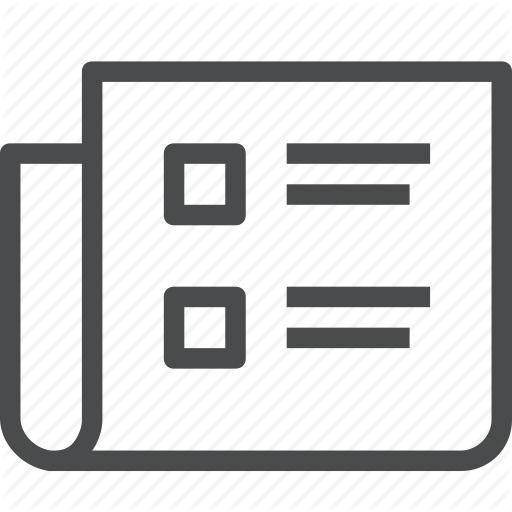
Fusion of deterministically generated photonic graph states
P. Thomas, L. Ruscio, O. Morin, G. Rempe
Nature 14 (2024).
Entanglement has evolved from an enigmatic concept of quantum physics to a key ingredient of quantum technology. It explains correlations between measurement outcomes that contradict classical physics and has been widely explored with small sets of individual qubits. Multi-partite entangled states build up in gate-based quantum-computing protocols and-from a broader perspective-were proposed as the main resource for measurement-based quantum-information processing 1,2 . The latter requires the ex-ante generation of a multi-qubit entangled state described by a graph 3-6 . Small graph states such as Bell or linear cluster states have been produced with photons 7-16 , but the proposed quantum-computing and quantum-networking applications require fusion of such states into larger and more powerful states in a programmable fashion 17-21 . Here we achieve this goal by using an optical resonator 22 containing two individually addressable atoms 23,24 . Ring 25 and tree 26 graph states with up to eight qubits, with the names reflecting the entanglement topology, are efficiently fused from the photonic states emitted by the individual atoms. The fusion process itself uses a cavity-assisted gate between the two atoms. Our technique is, in principle, scalable to even larger numbers of qubits and is the decisive step towards, for instance, a memory-less quantum repeater in a future quantum internet 27-29 . Using an optical resonator containing two individually addressable atoms in a single cavity, fusion of deterministically generated photonic graph states to create ring and tree graph states with up to eight qubits is demonstrated.
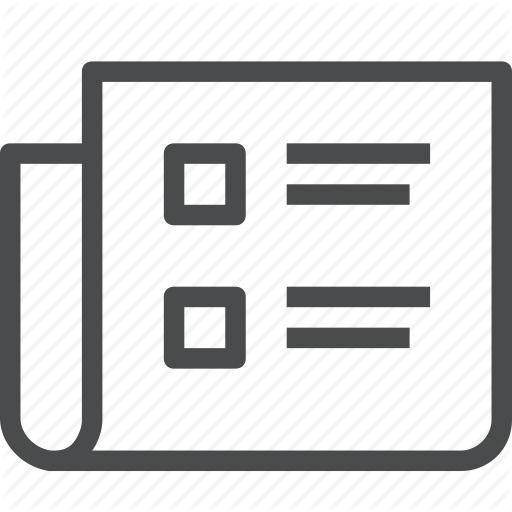
Electric-Field-Controlled Cold Dipolar Collisions between Trapped CH3F Molecules
M. Koller, F. Jung, J. Phrompao, M. Zeppenfeld, I. M. Rabey, G. Rempe
Physical Review Letters 128 (20), 203401 (2022).
Reaching high densities is a key step toward cold-collision experiments with polyatomic molecules. We use a cryofuge to load up to 2 x 10(7) CH3F molecules into a boxlike electric trap, achieving densities up to 10(7)/cm(3) at temperatures around 350 mK where the elastic dipolar cross section exceeds 7 x 10(-12) cm(2). We measure inelastic rate constants below 4 x 10(-8) cm(3)/s and control these by tuning a homogeneous electric field that covers a large fraction of the trap volume. Comparison to ab initio calculations gives excellent agreement with dipolar relaxation. Our techniques and findings are generic and immediately relevant for other cold-molecule collision experiments.
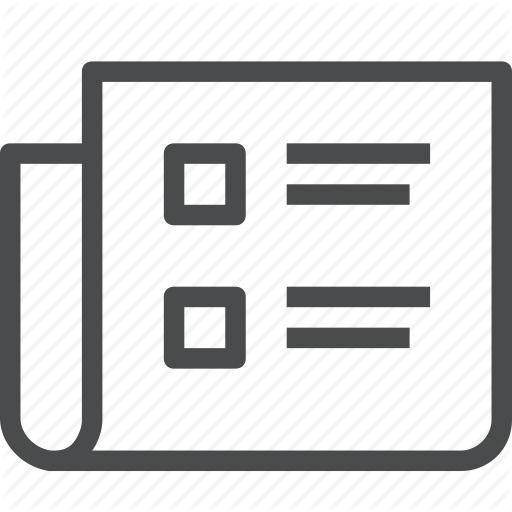
Quantum-Logic Gate between Two Optical Photons with an Average Efficiency above 40%
T. Stolz, H. Hegels, M. Winter, B. Rohr, Y. F. Hsiao, L. Husel, G. Rempe, S. Dürr
Physical Review X 12 (2), 21035 (2022).
Optical qubits uniquely combine information transfer in optical fibers with a good processing capability and are therefore attractive tools for quantum technologies. A large challenge, however, is to overcome the low efficiency of two-qubit logic gates. The experimentally achieved efficiency in an optical controlled NOT (cNoT) gate reached approximately 11% in 2003 and has seen no increase since. Here, we report on a new platform that was designed to surpass this long-standing record. The new scheme avoids inherently probabilistic protocols and, instead, combines aspects of two established quantum nonlinear systems: atom-cavity systems and Rydberg electromagnetically induced transparency. We demonstrate a CNOT gate between two optical photons with an average efficiency of 41.7(5)% at a postselected process fidelity of 81(2)%. Moreover, we extend the scheme to a CNOT gate with multiple target qubits and produce entangled states of presently up to five photons. All these achievements are promising and have the potential to advance optical quantum information processing in which almost all advanced protocols would profit from high-efficiency logic gates.
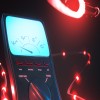
A nondestructive Bell-state measurement on two distant atomic qubits
S. Welte, P. Thomas, L. Hartung, S. Daiss, S. Langenfeld, O. Morin, G. Rempe, E. Distante
Nature Photonics 15 (7), 504-509 (2021).
One of the most fascinating aspects of quantum networks is their capability to distribute entanglement as a nonlocal communication resource(1). In a first step, this requires network-ready devices that can generate and store entangled states(2). Another crucial step, however, is to develop measurement techniques that allow for entanglement detection. Demonstrations for different platforms(3-13) suffer from being not complete, destructive or local. Here, we demonstrate a complete and nondestructive measurement scheme(14-16) that always projects any initial state of two spatially separated network nodes onto a maximally entangled state. Each node consists of an atom trapped inside an optical resonator from which two photons are successively reflected. Polarization measurements on the photons discriminate between the four maximally entangled states. Remarkably, such states are not destroyed by our measurement. In the future, our technique might serve to probe the decay of entanglement and to stabilize it against dephasing via repeated measurements(17,18).
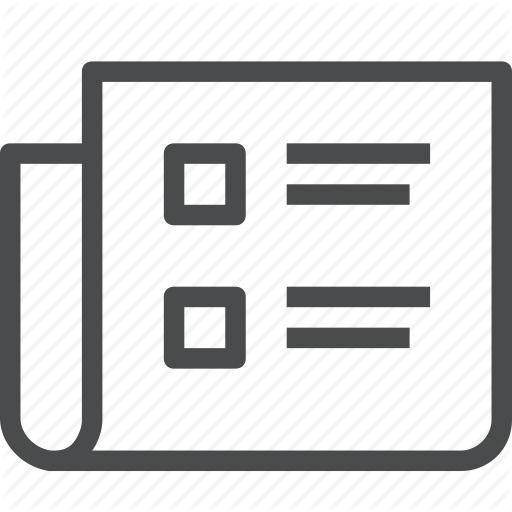
Detecting an Itinerant Optical Photon Twice without Destroying It
E. Distante, S. Daiss, S. Langenfeld, L. Hartung, P. Thomas, O. Morin, G. Rempe, S. Welte
Physical Review Letters 126 (25), 253603 (2021).
Nondestructive quantum measurements are central for quantum physics applications ranging from quantum sensing to quantum computing and quantum communication. Employing the toolbox of cavity quantum electrodynamics, we here concatenate two identical nondestructive photon detectors to repeatedly detect and track a single photon propagating through a 60 m long optical fiber. By demonstrating that the combined signal-to-noise ratio of the two detectors surpasses each single one by about 2 orders of magnitude, we experimentally verify a key practical benefit of cascaded nondemolition detectors compared to conventional absorbing devices.
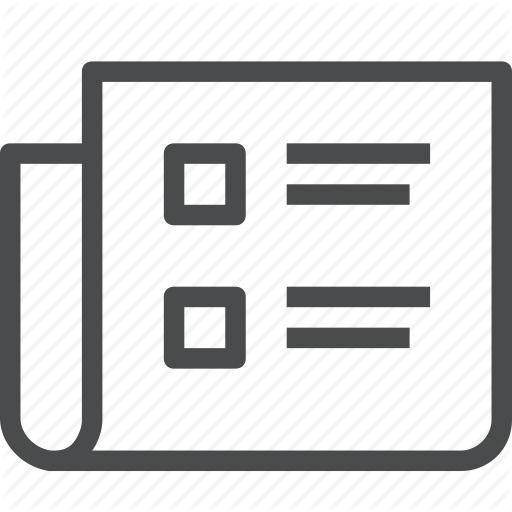
Quantum Repeater Node Demonstrating Unconditionally Secure Key Distribution
S. Langenfeld, P. Thomas, O. Morin, G. Rempe
Physical Review Letters 126 (23), 230506 (2021).
Long-distance quantum communication requires quantum repeaters to overcome photon loss in optical fibers. Here we demonstrate a repeater node with two memory atoms in an optical cavity. Both atoms are individually and repeatedly entangled with photons that are distributed until each communication partner has independently received one of them. An atomic Bell-state measurement followed by classical communication serves to establish a key. We demonstrate scaling advantage of the key rate, increase the effective attenuation length by a factor of 2, and beat the error-rate threshold of 11% for unconditionally secure communication, the corner stones for repeater-based quantum networks.
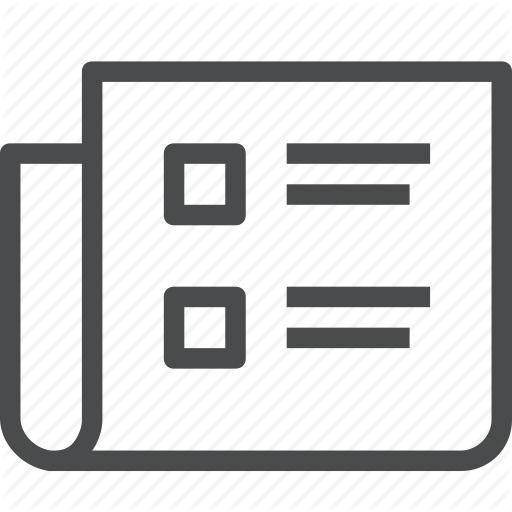
Quantensysteme lernen gemeinsames Rechnen
S. Daiss, G. Rempe
Physik in unserer Zeit (2021).
Quantencomputer besitzen heute erst wenige Qubits in einzelnen Aufbauten. Jetzt ist es gelungen, ein Quantengatter zwischen zwei Qubits in sechzig Metern Entfernung zu realisieren: ein Prototyp eines verteilt rechnenden Quantencomputers.
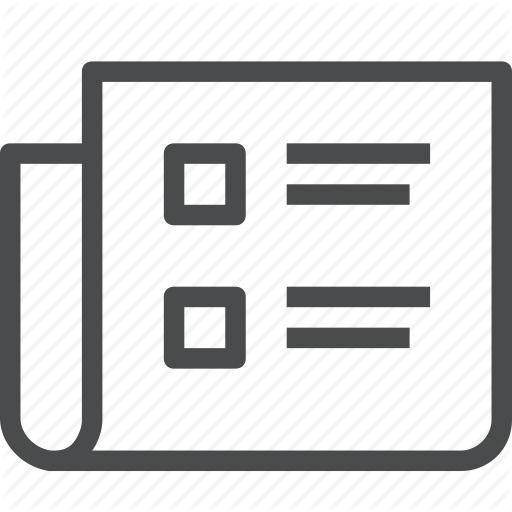
Quantum Teleportation between Remote Qubit Memories with Only a Single Photon as a Resource
S. Langenfeld, S. Welte, L. Hartung, S. Daiss, P. Thomas, O. Morin, E. Distante, G. Rempe
Physical Review Letters 126 (13), 130502 (2021).
Quantum teleportation enables the deterministic exchange of qubits via lossy channels. While it is commonly believed that unconditional teleportation requires a preshared entangled qubit pair, here we demonstrate a protocol that is in principle unconditional and requires only a single photon as an ex-ante prepared resource. The photon successively interacts, first, with the receiver and then with the sender qubit memory. Its detection, followed by classical communication, heralds a successful teleportation. We teleport six mutually unbiased qubit states with average fidelity (F) over bar= (88.3 +/- 1.3)% at a rate of 6 Hz over 60 m.
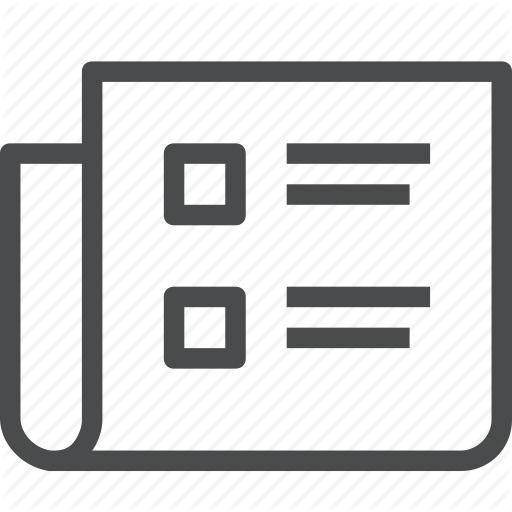
Continuous quantum light from a dark atom
K. N. Tolazzi, B. Wang, C. Ianzano, J. Neumeier, C. J. Villas-Boas, G. Rempe
Communications Physics 4 (1), 57 (2021).
Cycling processes are important in many areas of physics ranging from lasers to topological insulators, often offering surprising insights into dynamical and structural aspects of the respective system. Here we report on a quantum-nonlinear wave-mixing experiment where resonant lasers and an optical cavity define a closed cycle between several ground and excited states of a single atom. We show that, for strong atom-cavity coupling and steady-state driving, the entanglement between the atomic states and intracavity photon number suppresses the excited-state population via quantum interference, effectively reducing the cycle to the atomic ground states. The system dynamics then result from transitions within a harmonic ladder of entangled dark states, one for each cavity photon number, and a quantum Zeno blockade that generates antibunching in the photons emitted from the cavity. The reduced cycle suppresses unwanted optical pumping into atomic states outside the cycle, thereby enhancing the number of emitted photons. The ability of optically dark states to protect against decoherence makes them useful for the generation of entangled photons. Here, a continuous stream of single photons is generated by a controllable quantum Zeno effect between entangled atom-photon states.
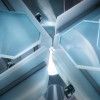
Nondestructive detection of photonic qubits
D. Niemietz, P. Farrera, S. Langenfeld, G. Rempe
Nature 591 (7851), 570-+ (2021).
One of the biggest challenges in experimental quantum information is to sustain the fragile superposition state of a qubit(1). Long lifetimes can be achieved for material qubit carriers as memories(2), at least in principle, but not for propagating photons that are rapidly lost by absorption, diffraction or scattering(3). The loss problem can be mitigated with a nondestructive photonic qubit detector that heralds the photon without destroying the encoded qubit. Such a detector is envisioned to facilitate protocols in which distributed tasks depend on the successful dissemination of photonic qubits(4,5), improve loss-sensitive qubit measurements(6,7) and enable certain quantum key distribution attacks(8). Here we demonstrate such a detector based on a single atom in two crossed fibre-based optical resonators, one for qubit-insensitive atom-photon coupling and the other for atomic-state detection(9). We achieve a nondestructive detection efficiency upon qubit survival of 79 +/- 3 per cent and a photon survival probability of 31 +/- 1 per cent, and we preserve the qubit information with a fidelity of 96.2 +/- 0.3 per cent. To illustrate the potential of our detector, we show that it can, with the current parameters, improve the rate and fidelity of long-distance entanglement and quantum state distribution compared to previous methods, provide resource optimization via qubit amplification and enable detection-loophole-free Bell tests.
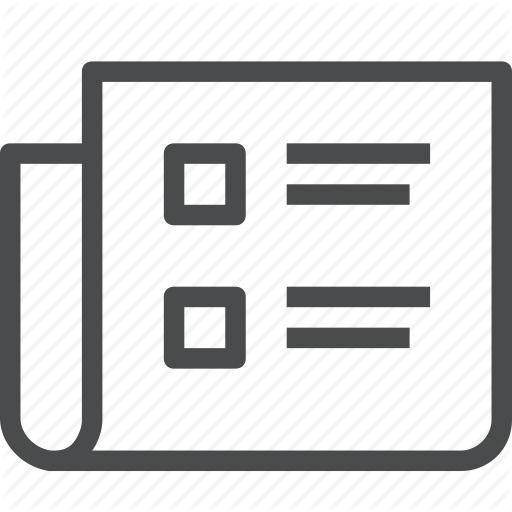
A quantum-logic gate between distant quantum-network modules
S. Daiss, S. Langenfeld, S. Welte, E. Distante, P. Thomas, L. Hartung, O. Morin, G. Rempe
Science 371 (6529), 614-+ (2021).
The big challenge in quantum computing is to realize scalable multi-qubit systems with cross-talk-free addressability and efficient coupling of arbitrarily selected qubits. Quantum networks promise a solution by integrating smaller qubit modules to a larger computing cluster. Such a distributed architecture, however, requires the capability to execute quantum-logic gates between distant qubits. Here we experimentally realize such a gate over a distance of 60 meters. We employ an ancillary photon that we successively reflect from two remote qubit modules, followed by a heralding photon detection, which triggers a final qubit rotation. We use the gate for remote entanglement creation of all four Bell states, Our nonlocal quantum-logic gate could be extended both to multiple qubits and many modules for a tailor-made multi-qubit computing register.
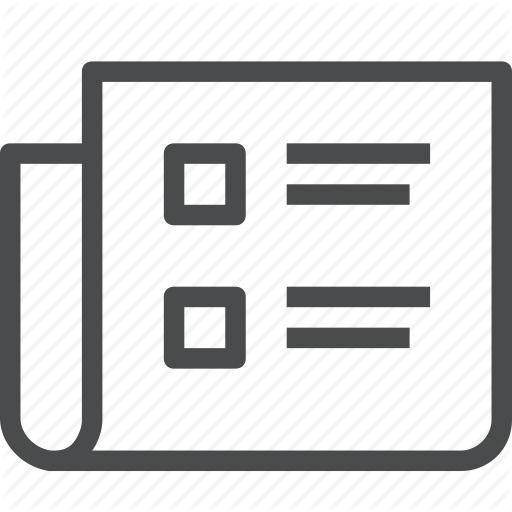
A network-ready random-access qubits memory
S. Langenfeld, O. Morin, M. Körber, G. Rempe
NPJ Quantum Information 6, 86 (2020).
Photonic qubits memories are essential ingredients of numerous quantum networking protocols. The ideal situation features quantum computing nodes that are efficiently connected to quantum communication channels via quantum interfaces. The nodes contain a set of long-lived matter qubits, the channels support the propagation of light qubits, and the interface couples light and matter qubits. Toward this vision, we here demonstrate a random-access multi-qubit write-read memory for photons using two rubidium atoms coupled to the same mode of an optical cavity, a setup that is known to feature quantum computing capabilities. We test the memory with more than ten independent photonic qubits, observe no noticeable cross-talk, and find no need for re-initialization even after ten write-read attempts. The combined write-read efficiency is 26% and the coherence time approaches 1 ms. With these features, the node constitutes a promising building block for a quantum repeater and ultimately a quantum internet.
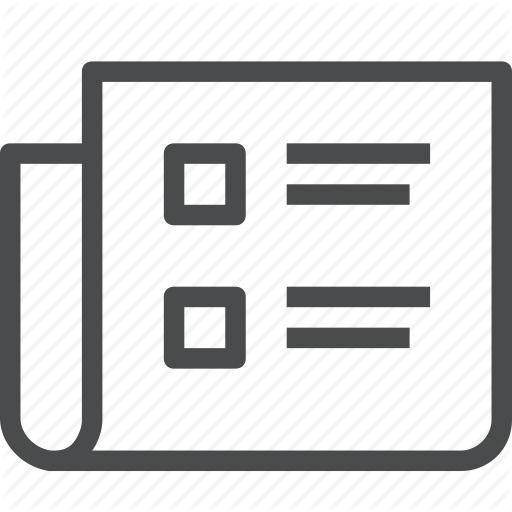
Buffer-gas cooling of molecules in the low-density regime: comparison between simulation and experiment
T. Gantner, M. Koller, X. Wu, G. Rempe, M. Zeppenfeld
Journal of Physics B 53, 14 (2020).
Cryogenic buffer gas cells have been a workhorse for the cooling of molecules in the last few decades. The straightforward sympathetic cooling principle makes them applicable to a huge variety of different species. Notwithstanding this success, detailed simulations of buffer gas cells are rare, and have never been compared to experimental data in the regime of low to intermediate buffer gas densities. Here, we present a numerical approach based on a trajectory analysis, with molecules performing a random walk in the cell due to collisions with a homogeneous buffer gas. This method can reproduce experimental flux and velocity distributions of molecules emerging from the buffer gas cell for varying buffer gas densities. This includes the strong decrease in molecule output from the cell for increasing buffer gas density and the so-called boosting effect, when molecules are accelerated by buffer-gas atoms after leaving the cell. The simulations provide various insights which could substantially improve buffer-gas cell design.
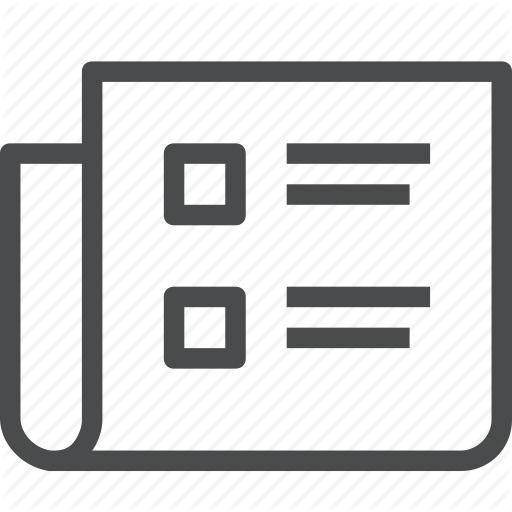
A quantum network node with crossed optical fibre cavities
M. Brekenfeld, D. Niemietz, J. D. Christesen, G. Rempe
Nature Physics 16 (6), 647-+ (2020).
A passive, heralded and high-fidelity quantum memory network node has been realized, which connects simultaneously to two quantum channels provided by orthogonally aligned optical fibre cavities coupled with a single atom. Quantum networks provide unique possibilities for resolving open questions on entanglement(1) and promise innovative applications ranging from secure communication to scalable computation(2). Although two quantum nodes coupled by a single channel are adequate for basic quantum communication tasks between two parties(3), fully functional large-scale quantum networks require a web-like architecture with multiply connected nodes(4). Efficient interfaces between network nodes and channels can be implemented with optical cavities(5). Using two optical fibre cavities coupled to one atom, we here realize a quantum network node that connects to two quantum channels, one provided by each cavity. It functions as a passive, heralded and high-fidelity quantum memory that requires neither amplitude- and phase-critical control fields(6-8) nor error-prone feedback loops(9). Our node is robust, fits naturally into larger fibre-based networks and has prospects for extensions including qubit-controlled quantum switches(10,11), routers(12,13) and repeaters(14,15).
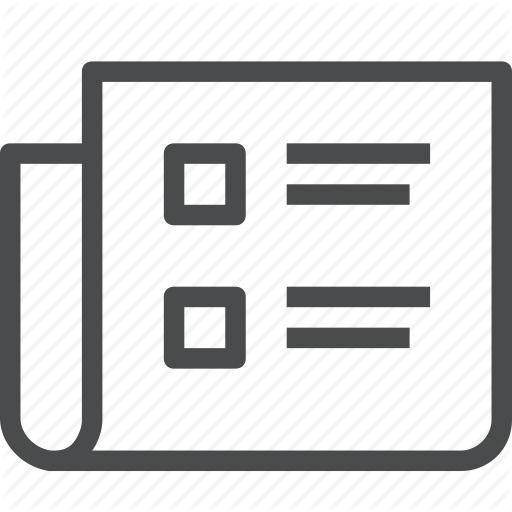
Continuous Generation of Quantum Light from a Single Ground-State Atom in an Optical Cavity
C. J. Villas-Boas, K. N. Tolazzi, B. Wang, C. Ianzano, G. Rempe
Physical Review Letters 124 (9), 93603 (2020).
We show an optical wave-mixing scheme that generates quantum light by means of a single three-level atom. The atom couples to an optical cavity and two laser fields that together drive a cycling current within the atom. Weak driving in combination with strong atom-cavity coupling induces transitions in a harmonic ladder of dark states, accompanied by single-photon emission via a quantum Zeno effect and suppression of atomic excitation via quantum interference. For strong driving, the system can generate coherent or Schrodinger cat-like fields with frequencies distinct from those of the applied lasers.
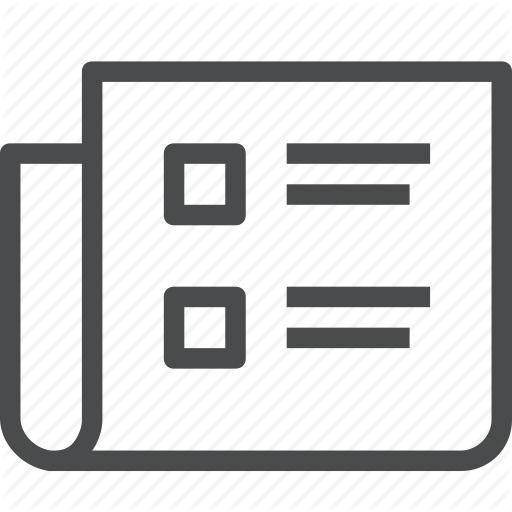
Accurate photonic temporal mode analysis with reduced resources
O. Morin, S. Langenfeld, M. Korber, G. Rempe
Physical Review A 101 (1), 13801 (2020).
The knowledge and thus characterization of the temporal modes of quantum light fields is important in many areas of quantum physics ranging from experimental setup diagnosis to fundamental-physics investigations. Recent results showed how the autocorrelation function computed from continuous-wave homodyne measurements can be a powerful way to access the temporal mode structure. Here, we push forward this method by providing a deeper understanding and by showing how to extract the amplitude and phase of the temporal mode function with reduced experimental resources. Moreover, a quantitative analysis allows us to identify a regime of parameters where the method provides a trustworthy reconstruction, which we illustrate experimentally.
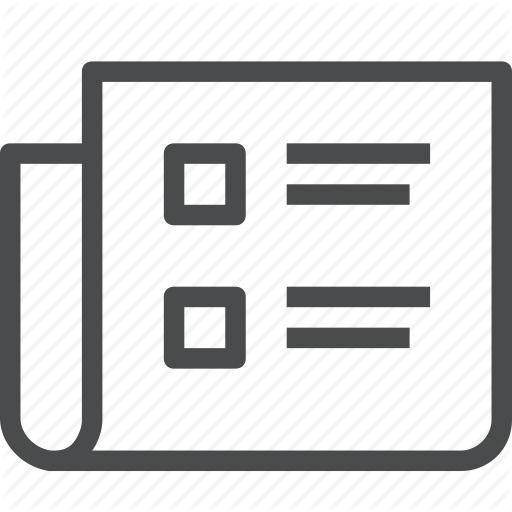
Dark-time decay of the retrieval efficiency of light stored as a Rydberg excitation in a noninteracting ultracold gas
S. Schmidt-Eberle, T. Stolz, G. Rempe, S. Dürr
Physical Review A 101 (1), 13421 (2020).
We study the dark-time decay of the retrieval efficiency for light stored in a Rydberg state in an ultracold gas of Rb-87 atoms based on electromagnetically induced transparency (EIT). Using low atomic density to avoid dephasing caused by atom-atom interactions, we measure a 1/e time of 30 mu s for the 80S state in free expansion. One of the dominant limitations is the combination of photon recoil and thermal atomic motion at 0.2 mu K. If the 1064-nm dipole trap is left on, then the 1/e time is reduced to 13 mu s, in agreement with a model taking differential light shifts and gravitational sag into account. To characterize how coherent the retrieved light is, we overlap it with reference light and measure the visibility V of the resulting interference pattern, obtaining V > 90% for short dark time. Our experimental work is accompanied by a detailed model for the dark-time decay of the retrieval efficiency of light stored in atomic ensembles. The model is generally applicable for photon storage in Dicke states, such as in EIT with A-type or ladder-type level schemes and in Duan-Lukin-Cirac-Zoller singlephoton sources. The model includes a treatment of the dephasing caused by thermal atomic motion combined with net photon recoil, as well as the influence of trapping potentials. It takes into account that the signal light field is typically not a plane wave. The model maps the retrieval efficiency to single-atom properties and shows that the retrieval efficiency is related to the decay of fringe visibility in Ramsey spectroscopy and to the spatial first-order coherence function of the gas.
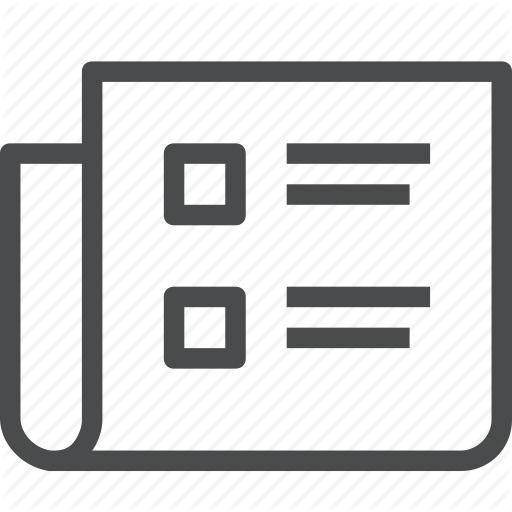
Deterministic Shaping and Reshaping of Single-Photon Temporal Wave Functions
O. Morin, M. Korber, S. Langenfeld, G. Rempe
Physical Review Letters 123 (13), 133602 (2019).
Thorough control of the optical mode of a single photon is essential for quantum information applications. We present a comprehensive experimental and theoretical study of a light-matter interface based on cavity quantum electrodynamics. We identify key parameters like the phases of the involved light fields and demonstrate absolute, flexible, and accurate control of the time-dependent complex-valued wave function of a single photon over several orders of magnitude. This capability will be an important tool for the development of distributed quantum systems with multiple components that interact via photons.