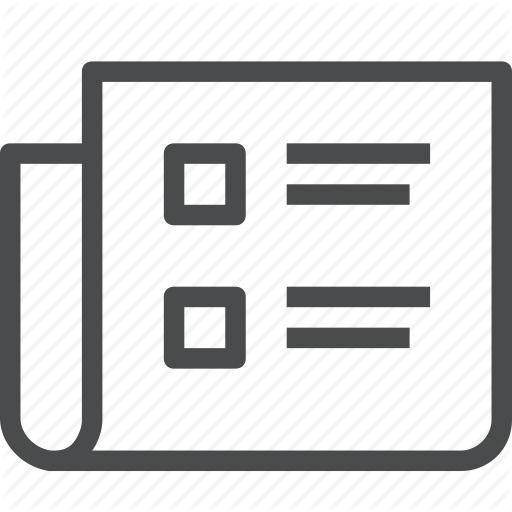
Distinguishing an Anderson insulator from a many-body localized phase through space-time snapshots with neural networks
F. Kotthoff, F. Pollmann, G. De Tomasi
Physical Review B 104, 224307 (2021).
Distinguishing the dynamics of an Anderson insulator from a many-body localized (MBL) phase is an experimentally challenging task. In this work we propose a method based on machine learning techniques to analyze experimental snapshot data to separate the two phases. We show how to train three-dimensional convolutional neural networks (CNNs) using space-time Fock-state snapshots, allowing us to obtain dynamic information about the system. We benchmark our method on a paradigmatic model showing MBL (t-V model with quenched disorder), where we obtain a classification accuracy of approximate to 80% between an Anderson insulator and an MBL phase. We underline the importance of providing temporal information to the CNNs and we show that CNNs learn the crucial difference between an Anderson localized and an MBL phase, namely the difference in the propagation of quantum correlations. Particularly, we show that the misclassified MBL samples are characterized by an unusually slow propagation of quantum correlations, and thus the CNNs label them wrongly as Anderson localized. Finally, we apply our method to the case with quasiperiodic potential, known as the Aubry-Andre model (AA model). We find that the CNNs have more difficulties in separating the two phases. We show that these difficulties are due to the fact that the MBL phase of the AA model is characterized by a slower information propagation for numerically accessible system sizes.
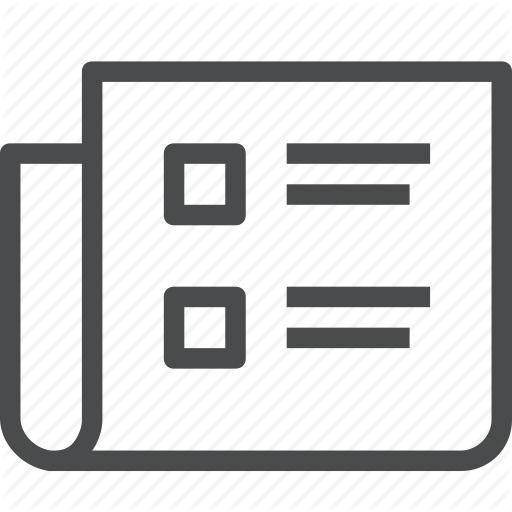
Gapless Topological Phases and Symmetry-Enriched Quantum Criticality
R. Verresen, R. Thorngren, N.G. Jones, F. Pollmann
Physical Review X 11, 041059 (2021).
We introduce topological invariants for gapless systems and study the associated boundary phenomena. More generally, the symmetry properties of the low-energy conformal field theory (CFT) provide discrete invariants establishing the notion of symmetry-enriched quantum criticality. The charges of nonlocal scaling operators, or more generally, of symmetry defects, are topological and imply the presence of localized edge modes. We primarily focus on the 1 + 1d case where the edge has a topological degeneracy, whose finite-size splitting can be exponential or algebraic in system size depending on the involvement of additional gapped sectors. An example of the exponential case is given by tuning the spin-1 Heisenberg chain to a symmetry-breaking Ising phase. An example of the algebraic case arises between the gapped Ising and cluster phases: This symmetry-enriched Ising CFT has an edge mode with finite-size splitting scaling as 1/L14. In addition to such new cases, our formalism unifies various examples previously studied in the literature. Similar to gapped symmetry-protected topological phases, a given CFT can split into several distinct symmetry-enriched CFTs. This raises the question of classification, to which we give a partial answer-including a complete characterization of symmetry-enriched 1 + 1d Ising CFTs. Nontrivial topological invariants can also be constructed in higher dimensions, which we illustrate for a symmetry-enriched 2 + 1d CFT without gapped sectors.
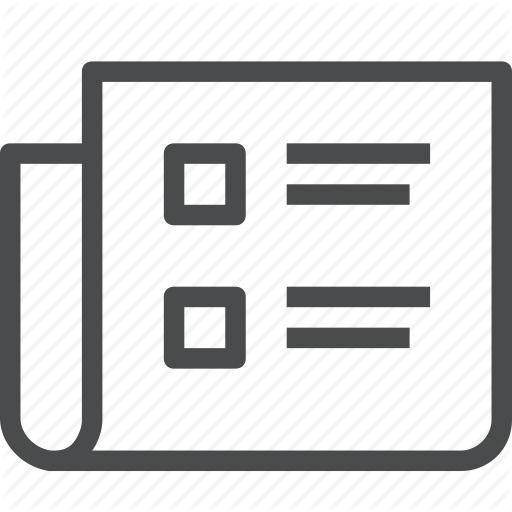
Realizing topologically ordered states on a quantum processor
K. J. Satzinger, Y. Liu, A. Smith, C. Knapp, M. Newman, C. Jones, Z. Chen, C. Quintana, X. Mi, A. Dunsworth, C. Gidney, I. Aleiner, F. Arute, K. Arya, J. Atalaya, R. Babbush, J. C. Bardin, R. Barends, J. Basso, A. Bengtsson, A. Bilmes, M. Broughton, B. B. Buckley, D. A. Buell, B. Burkett, N. Bushnell, B. Chiaro, R. Collins, W. Courtney, S. Demura, A. R. Derk, D. Eppens, C. Erickson, E. Farhi, L. Foaro, A. G. Fowler, B. Foxen, M. Giustina, A. Greene, J. A. Gross, M. P. Harrigan, S. D. Harrington, J. Hilton, S. Hong, T. Huang, W. J. Huggins, L. B. Ioffe, S. V. Isakov, E. Jeffrey, Z. Jiang, D. Kafri, K. Kechedzhi, T. Khattar, S. Kim, P. V. Klimov, A.N. Korotkov, F. Kostritsa, D. Landhuis, P. Laptev, A. Locharla, E. Lucero, O. Martin, J. R. McClean, M. McEwen, K. C. Miao, M. Mohseni, S. Montazeri, W. Mruczkiewicz, J. Mutus, O. Naaman, M. Neeley, C. Neill, M. Y. Niu, T. E. O'Brien, A. Opremcak, B. Pató, A. Petukhov, N. C. Rubin, D. Sank, V. Shvarts, D. Strain, M. Szalay, B. Villalonga, T. C. White, Z. Yao, P. Yeh, J. Yoo, A. Zalcman, H. Neven, S. Boixo, A. Megrant, Y. Chen, J. Kelly, V. Smelyanskiy, A. Kitaev, M. Knap, F. Pollmann, P. Roushan
Science 374, 1237-1241 (2021).
The discovery of topological order has revised the understanding of quantum matter and provided the theoretical foundation for many quantum error-correcting codes. Realizing topologically ordered states has proven to be challenging in both condensed matter and synthetic quantum systems. We prepared the ground state of the toric code Hamiltonian using an efficient quantum circuit on a superconducting quantum processor. We measured a topological entanglement entropy near the expected value of -ln2 and simulated anyon interferometry to extract the braiding statistics of the emergent excitations. Furthermore, we investigated key aspects of the surface code, including logical state injection and the decay of the nonlocal order parameter. Our results demonstrate the potential for quantum processors to provide insights into topological quantum matter and quantum error correction.
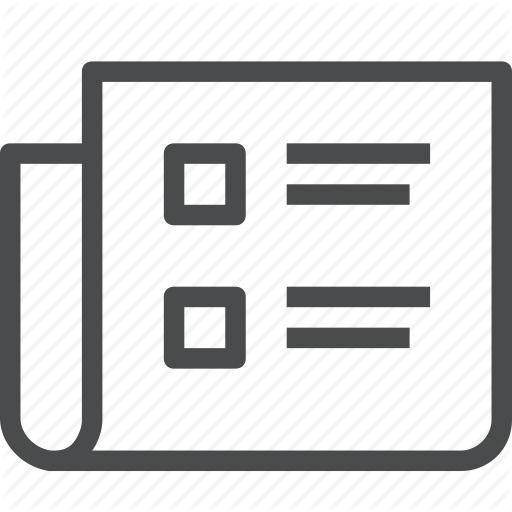
Dynamics of Negativity of a Wannier-Stark Many-Body Localized System Coupled to a Bath
E. Wybo, M. Knap, F. Pollmann
Physica Status Solidi B-Basic Solid State Physics 2100161 (2021).
An interacting system subjected to a strong linear potential can host a many-body localized (MBL) phase when being slightly perturbed. This so-called Wannier-Stark or "tilted-field" MBL phase inherits many properties from the well-investigated disordered MBL phase, and provides an alternative route to experimentally engineer interacting localized systems without quenched disorder. Herein, the dynamics of entanglement in a Wannier-Stark MBL system coupled to a dephasing environment is investigated. As an accessible entanglement proxy, the third Renyi negativity R 3 is used, which reduces to the third Renyi entropy in case the system is isolated from the environment. This measure captures the characteristic logarithmic growth of interacting localized phases in the intermediate-time regime, where the effects of the coupling to the environment are not yet dominating the dynamics. Thus, it forms a tool to distinguish Wannier-Stark MBL from noninteracting Wannier-Stark localization up to intermediate time-scales, and to quantify quantum correlations in mixed-state dynamics.
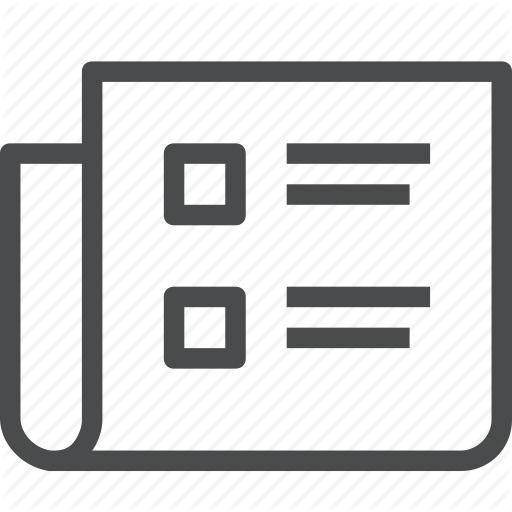
Skeleton of matrix-product-state-solvable models connecting topological phases of matter
N.G. Jones, J. Bibo, B. Jobst, F. Pollmann, A. Smith, R. Verresen
Physical Review Research 3, 033265 (2021).
Models whose ground states can be written as an exact matrix-product state (MPS) provide valuable insights into phases of matter. While MPS-solvable models are typically studied as isolated points in a phase diagram, they can belong to a connected network of MPS-solvable models, which we call the MPS skeleton. As a case study where we can completely unearth this skeleton, we focus on the one-dimensional BDI class-noninteracting spinless fermions with time-reversal symmetry. This class, labeled by a topological winding number, contains the Kitaev chain and is Jordan-Wigner-dual to various symmetry-breaking and symmetry-protected topological (SPT) spin chains. We show that one can read off from the Hamiltonian whether its ground state is an MPS: defining a polynomial whose coefficients are the Hamiltonian parameters, MPS-solvability corresponds to this polynomial being a perfect square. We provide an explicit construction of the ground state MPS, its bond dimension growing exponentially with the range of the Hamiltonian. This complete characterization of the MPS skeleton in parameter space has three significant consequences: (i) any two topologically distinct phases in this class admit a path of MPS-solvable models between them, including the phase transition which obeys an area law for its entanglement entropy; (ii) we illustrate that the subset of MPS-solvable models is dense in this class by constructing a sequence of MPS-solvable models which converge to the Kitaev chain (equivalently, the quantum Ising chain in a transverse field); (iii) a subset of these MPS states can be particularly efficiently processed on a noisy intermediate-scale quantum computer.
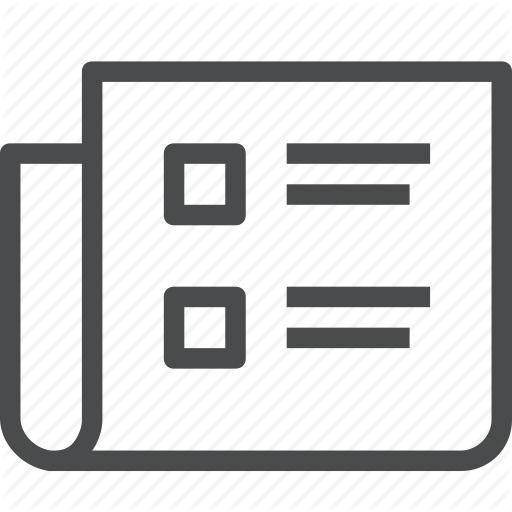
Signatures of Quantum Phase Transitions after Quenches in Quantum Chaotic One-Dimensional Systems
A. Haldar, K. Mallayya, M. Heyl, F. Pollmann, M. Rigol, A. Das
Physical Review X 11, 031062 (2021).
Quantum phase transitions are central to our understanding of why matter at very low temperatures can exhibit starkly different properties upon small changes of microscopic parameters. Accurately locating those transitions is challenging experimentally and theoretically. Here, we show that the antithetic strategy of forcing systems out of equilibrium via sudden quenches provides a route to locate quantum phase transitions. Specifically, we show that such transitions imprint distinctive features in the intermediate-time dynamics, and results after equilibration, of local observables in quantum chaotic spin chains. Furthermore, we show that the effective temperature in the expected thermal-like states after equilibration can exhibit minima in the vicinity of the quantum critical points. We discuss how to test our results in experiments with Rydberg atoms and explore nonequilibrium signatures of quantum critical points in models with topological transitions.
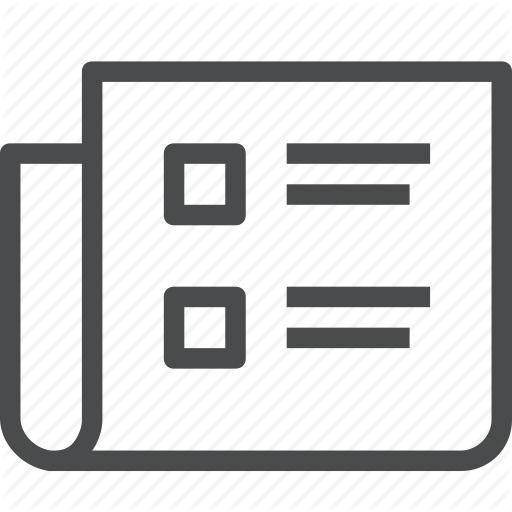
Observing non-ergodicity due to kinetic constraints in tilted Fermi-Hubbard chains
S. Scherg, T. Kohlert, P. Sala, F. Pollmann, H.M. Bharath, I. Bloch, M. Aidelsburger
Nature Communications 12, 4490 (2021).
The thermalization of isolated quantum many-body systems is deeply related to fundamental questions of quantum information theory. While integrable or many-body localized systems display non-ergodic behavior due to extensively many conserved quantities, recent theoretical studies have identified a rich variety of more exotic phenomena in between these two extreme limits. The tilted one-dimensional Fermi-Hubbard model, which is readily accessible in experiments with ultracold atoms, emerged as an intriguing playground to study non-ergodic behavior in a clean disorder-free system. While non-ergodic behavior was established theoretically in certain limiting cases, there is no complete understanding of the complex thermalization properties of this model. In this work, we experimentally study the relaxation of an initial charge-density wave and find a remarkably long-lived initial-state memory over a wide range of parameters. Our observations are well reproduced by numerical simulations of a clean system. Using analytical calculations we further provide a detailed microscopic understanding of this behavior, which can be attributed to emergent kinetic constraints.
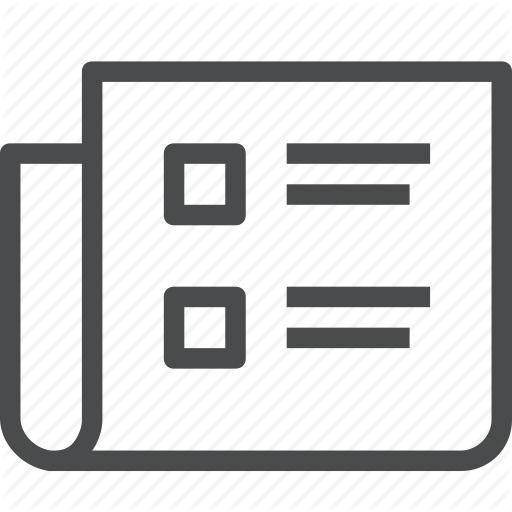
Rare thermal bubbles at the many-body localization transition from the Fock space point of view
G. De Tomasi, I.M. Khaymovich, F. Pollmann, S. Warzel
Physical Review B 104, 024202 (2021).
In this paper we study the many-body localization (MBL) transition and relate it to the eigenstate structure in the Fock space. Besides the standard entanglement and multifractal probes, we introduce the radial probability distribution of eigenstate coefficients with respect to the Hamming distance in the Fock space and relate the cumulants of this distribution to the properties of the quasilocal integrals of motion in the MBL phase. We demonstrate nonself-averaging property of the many-body fractal dimension Dq and directly relate it to the jump of Dq as well as of the localization length of the integrals of motion at the MBL transition. We provide an example of the continuous many-body transition confirming the above relation via the self-averaging of Dq in the whole range of parameters. Introducing a simple toy model, which hosts ergodic thermal bubbles, we give analytical evidences both in standard probes and in terms of newly introduced radial probability distribution that the MBL transition in the Fock space is consistent with the avalanche mechanism for delocalization, i.e., the Kosterlitz-Thouless scenario. Thus, we show that the MBL transition can been seen as a transition between ergodic states to nonergodic extended states and put the upper bound for the disorder scaling for the genuine Anderson localization transition with respect to the noninteracting case.
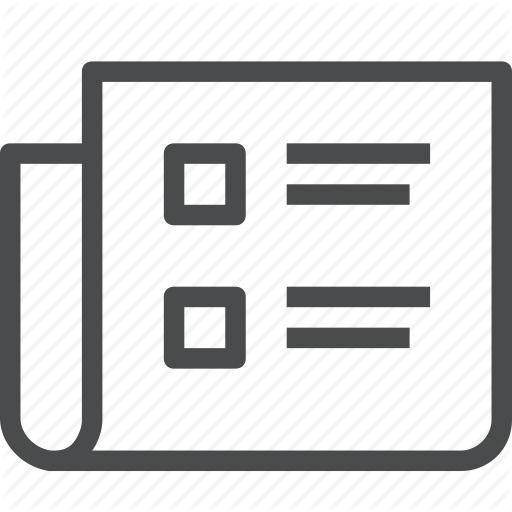
The quantum sine-Gordon model with quantum circuits
A. Roy, D. Schuricht, J. Hauschild, F. Pollmann, H. Saleur
Nuclear Physics B 968, 115445 (2021).
Analog quantum simulation has the potential to be an indispensable technique in the investigation of complex quantum systems. In this work, we numerically investigate a one-dimensional, faithful, analog, quantum electronic circuit simulator built out of Josephson junctions for one of the paradigmatic models of an integrable quantum field theory: the quantum sine-Gordon (qSG) model in 1+1 space-time dimensions. We analyze the lattice model using the density matrix renormalization group technique and benchmark our numerical results with existing Bethe ansatz computations. Furthermore, we perform analytical form-factor calculations for the two-point correlation function of vertex operators, which closely agree with our numerical computations. Finally, we compute the entanglement spectrum of the qSG model. We compare our results with those obtained using the integrable lattice-regularization based on the quantum XYZ chain and show that the quantum circuit model is less susceptible to corrections to scaling compared to the XYZ chain. We provide numerical evidence that the parameters required to realize the qSG model are accessible with modern-day superconducting circuit technology, thus providing additional credence towards the viability of the latter platform for simulating strongly interacting quantum field theories. (C) 2021 The Author(s). Published by Elsevier B.V.
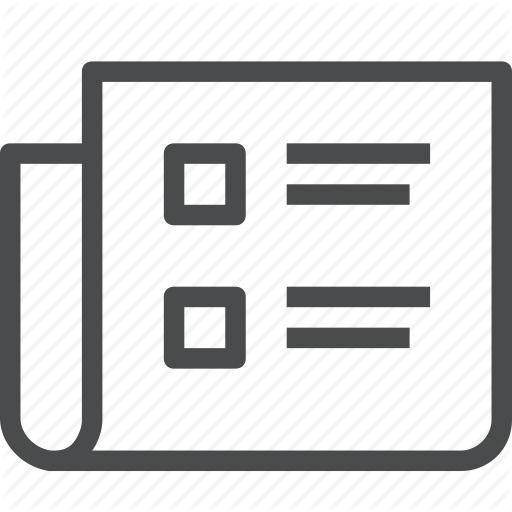
Parallel quantum simulation of large systems on small NISQ computers
F. Barratt, J. Dborin, M. Bal, V. Stojevic, F. Pollmann, A.G. Green
Npj Quantum Information 7, 79 (2021).
Tensor networks permit computational and entanglement resources to be concentrated in interesting regions of Hilbert space. Implemented on NISQ machines they allow simulation of quantum systems that are much larger than the computational machine itself. This is achieved by parallelising the quantum simulation. Here, we demonstrate this in the simplest case; an infinite, translationally invariant quantum spin chain. We provide Cirq and Qiskit code that translates infinite, translationally invariant matrix product state (iMPS) algorithms to finite-depth quantum circuit machines, allowing the representation, optimisation and evolution of arbitrary one-dimensional systems. The illustrative simulated output of these codes for achievable circuit sizes is given.
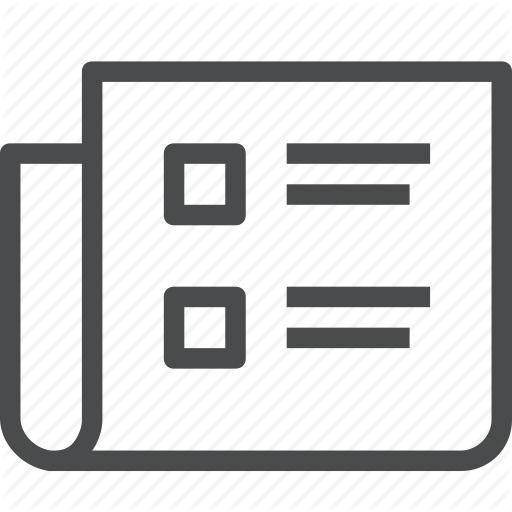
Entanglement growth in diffusive systems with large spin
T. Rakovszky, F. Pollmann, C. von Keyserlingk
Communications Physics 4, 91 (2021).
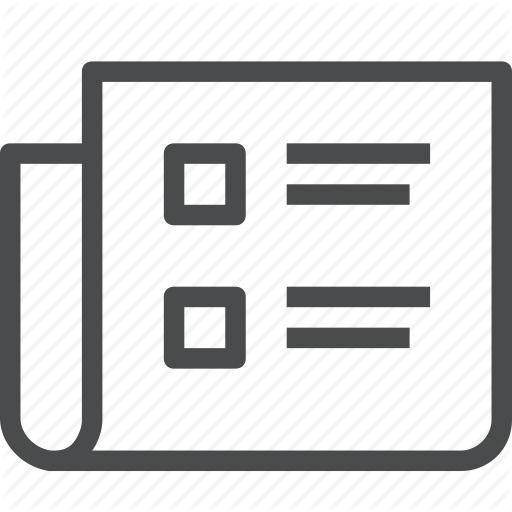
Emergent fracton dynamics in a nonplanar dimer model
J. Feldmeier, F. Pollmann, M. Knap
Physical Review B 103 (9), 94303 (2021).
We study the late time relaxation dynamics of a pure U(1) lattice gauge theory in the form of a dimer model on a bilayer geometry. To this end, we first develop a proper notion of hydrodynamic transport in such a system by constructing a global conservation law that can be attributed to the presence of topological solitons. The correlation functions of local objects charged under this conservation law can then be used to study the universal properties of the dynamics at late times, applicable to both quantum and classical systems. Performing the time evolution via classically simulable automata circuits unveils a rich phenomenology of the system's nonequilibrium properties: For a large class of relevant initial states, local charges are effectively restricted to move along one-dimensional “tubes” within the quasi-two-dimensional system, displaying fracton-like mobility constraints. The timescale on which these tubes are stable diverges with increasing systems size, yielding a novel mechanism for nonergodic behavior in the thermodynamic limit. We further explore the role of geometry by studying the system in a quasi-one-dimensional limit, where the Hilbert space is strongly fragmented due to the emergence of an extensive number of conserved quantities. This provides an instance of a recently introduced concept of “statistically localized integrals of motion,” whose universal anomalous hydrodynamics we determine by a mapping to a problem of classical tracer diffusion. We conclude by discussing how our approach might generalize to study transport in other lattice gauge theories.
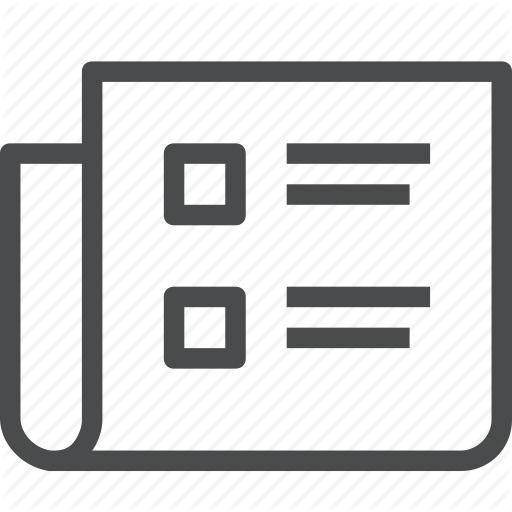
Real- and Imaginary-Time Evolution with Compressed Quantum Circuits
S.-H. Lin, R. Dilip, A.G. Green, A. Smith, F. Pollmann
Prx Quantum 2, 010342 (2021).
The current generation of noisy intermediate-scale quantum computers introduces new opportunities to study quantum many-body systems. In this paper, we show that quantum circuits can provide a dramatically more efficient representation than current classical numerics of the quantum states generated under nonequilibrium quantum dynamics. For quantum circuits, we perform both real- and imaginary-time evolution using an optimization algorithm that is feasible on near-term quantum computers. We benchmark the algorithms by finding the ground state and simulating a global quench of the transverse-field Ising model with a longitudinal field on a classical computer. Furthermore, we implement (classically optimized) gates on a quantum processing unit and demonstrate that our algorithm effectively captures real-time evolution.
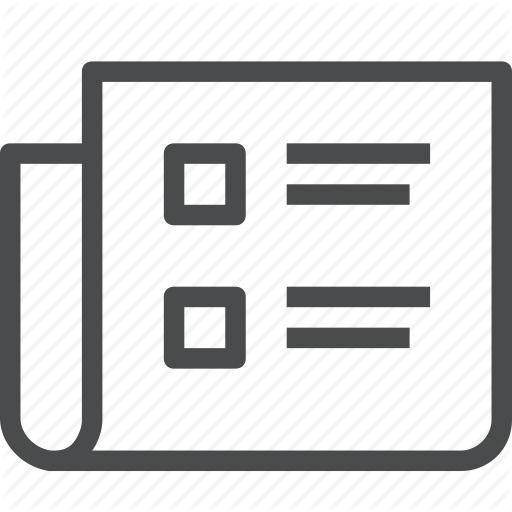
Visualizing quasiparticles from quantum entanglement for general one-dimensional phases
E. Wybo, F. Pollmann, S.L. Sondhi, Y. You
Physical Review B 103, 115120 (2021).
In this paper, we present a quantum information framework for the entanglement behavior of the low-energy quasiparticle (QP) excitations in various quantum phases in one-dimensional (1D) systems. We first establish an exact correspondence between the correlation matrix and the QP entanglement Hamiltonian for free fermions and find an extended in-gap state in the QP entanglement Hamiltonian as a consequence of the position uncertainty of the QP. A more general understanding of such an in-gap state can be extended to a Kramers theorem for the QP entanglement Hamiltonian, which also applies to strongly interacting systems. Further, we present a set of ubiquitous entanglement spectrum features, dubbed entanglement fragmentation, conditional mutual information, and measurement-induced nonlocal entanglement for QPs in 1D symmetry protected topological phases. Our result thus provides another framework to identify different phases of matter in terms of their QP entanglement.
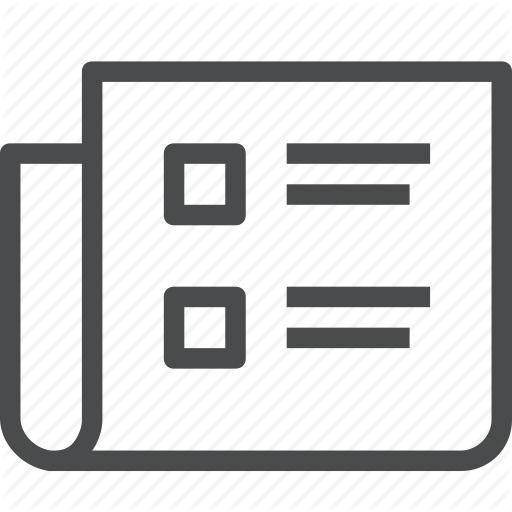
Anomalous Diffusion in Dipole- and Higher-Moment-Conserving Systems
J. Feldmeier, P. Sala, G. De Tomasi, F. Pollmann, M. Knap
Physical Review Letters 125 (24), 245303 (2020).
The presence of global conserved quantities in interacting systems generically leads to diffusive transport at late times. Here, we show that systems conserving the dipole moment of an associated global charge, or even higher-moment generalizations thereof, escape this scenario, displaying subdiffusive decay instead. Modeling the time evolution as cellular automata for specific cases of dipole- and quadrupole conservation, we numerically find distinct anomalous exponents of the late time relaxation. We explain these findings by analytically constructing a general hydrodynamic model that results in a series of exponents depending on the number of conserved moments, yielding an accurate description of the scaling form of charge correlation functions. We analyze the spatial profile of the correlations and discuss potential experimentally relevant signatures of higher-moment conservation.
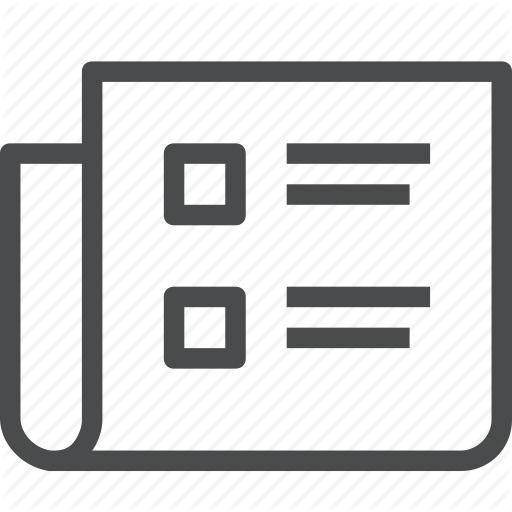
Experimental probes of Stark many-body localization
S.R. Taylor, M. Schulz, F. Pollmann, R. Moessner
Physical Review B 102 (5), 054206 (2020).
Recent work has focused on exploring many-body localization (MBL) in systems without quenched disorder: one such proposal is Stark MBL in which small perturbations to a strong linear potential yield localization. However, as with conventional MBL, it is challenging to experimentally distinguish between noninteracting localization and true MBL. In this paper, we show that several existing experimental probes, designed specifically to differentiate between these scenarios, work similarly in the Stark MBL setting. In particular, we show that a modified spin-echo response shows clear signs of a power-law decay for Stark MBL while quickly saturating for disorder-free Wannier-Stark localization. Furthermore, we observe the characteristic logarithmic-in-time spreading of quantum mutual information in the Stark MBL regime, and an absence of spreading in a noninteracting Stark-localized system. We also show that there are no significant differences in several existing MBL measures for a system consisting of soft-core bosons with repulsive on-site interactions. Lastly, we discuss why curvature or small disorder are needed for an accurate reproduction of MBL phenomenology and how this may be illustrated in experiment. This also connects with recent progress on Hilbert space fragmentation in "fractonic" models with a conserved dipole moment, and we suggest this as an auspicious platform for experimental investigations of these phenomena.
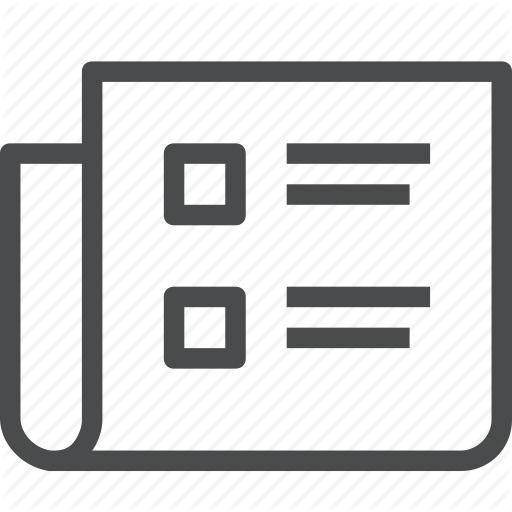
Entanglement dynamics of a many-body localized system coupled to a bath
E. Wybo, M. Knap, F. Pollmann
Physical Review B 102 (6), 064303 (2020).
The combination of strong disorder and interactions in closed quantum systems can lead to many-body localization (MBL). However, this quantum phase is not stable when the system is coupled to a thermal environment. We investigate how MBL is destroyed in systems that are weakly coupled to a dephasive Markovian environment by focusing on their entanglement dynamics. We numerically study the third Renyi negativity R-3, a recently proposed entanglement proxy based on the negativity that captures the unbounded logarithmic growth in the closed case and that can be computed efficiently with tensor networks. We also show that the decay of R-3 follows a stretched exponential law, similarly to the imbalance, with, however, a smaller stretching exponent.
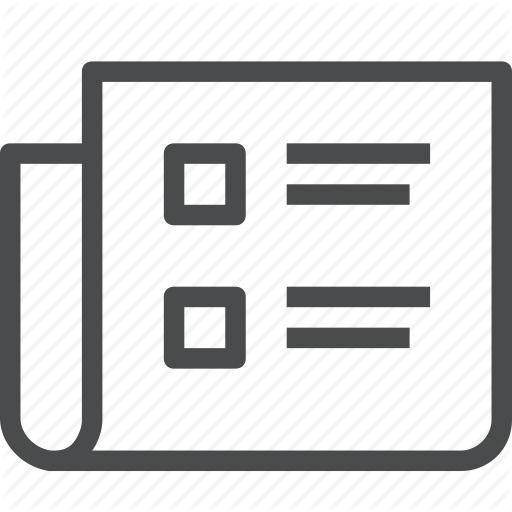
Higher-order entanglement and many-body invariants for higher-order topological phases
Y. You, J. Bibo, F. Pollmann
Physical Review Research 2, 33192 (2020).
We discuss how strongly interacting higher-order symmetry protected topological (HOSPT) phases can be characterized from the entanglement perspective: First, we introduce a topological many-body invariant which reveals the noncommutative algebra between a flux operator and Cn rotations. We argue that this invariant denotes the angular momentum carried by the instanton which is closely related to the discrete Wen-Zee response and the fractional corner charge. Second, we define a new entanglement property, dubbed “higher-order entanglement,” to scrutinize and differentiate various higher-order topological phases from a hierarchical sequence of the entanglement structure. We support our claims by numerically studying a super-lattice Bose-Hubbard model that exhibits different HOSPT phases.
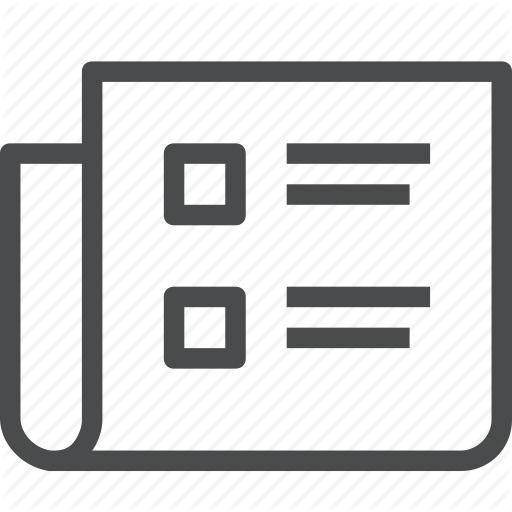
Entanglement Hamiltonian of the 1+1-dimensional free, compactified boson conformal field theory
A. Roy, F. Pollmann, H, Saleur
Journal of Statistical Mechanics - Theory and Experiment 2020 (8), 083104 (2020).
Entanglement or modular Hamiltonians play a crucial role in the investigation of correlations in quantum field theories. In particular, in 1 + 1 space-time dimensions, the spectra of entanglement Hamiltonians of conformal field theories (CFTs) for certain geometries are related to the spectra of the physical Hamiltonians of corresponding boundary CFTs. As a result, conformal invariance allows exact computation of the spectra of the entanglement Hamiltonians for these models. In this work, we perform this computation of the spectrum of the entanglement Hamiltonian for the free compactified boson CFT over a finite spatial interval. We compare the analytical results obtained for the continuum theory with numerical simulations of a lattice-regularized model for the CFT using density matrix renormalization group technique. To that end, we use a lattice regularization provided by superconducting quantum electronic circuits, built out of Josephson junctions and capacitors. Up to non-universal effects arising due to the lattice regularization, the numerical results are compatible with the predictions of the exact computations.
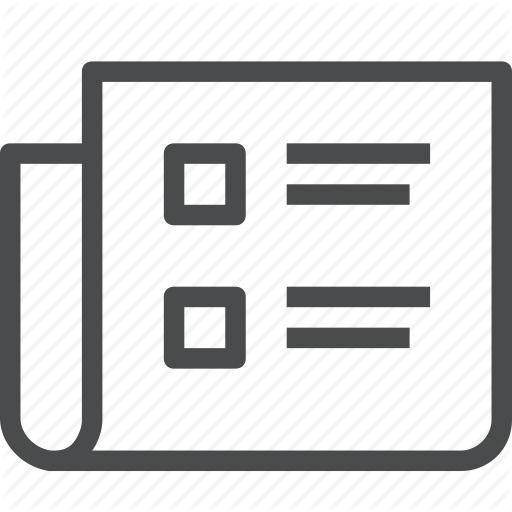
Fractional corner charges in a 2D super-lattice Bose-Hubbard model
J. Bibo, I. Lovas, Y. You, F. Grusdt, F. Pollmann
Physical Review B 102, 041126 (R) (2020).
We study higher order topology in the presence of strong interactions in a two-dimensional, experimentally accessible superlattice Bose-Hubbard model with alternating hoppings and strong on-site repulsion. We evaluate the phase diagram of the model around half-filling using the density renormalization group ansatz and find two gapped phases separated by a gapless superfluid region. We demonstrate that the gapped states realize two distinct higher order symmetry protected topological phases, which are protected by a combination of charge conservation and C4 lattice symmetry. The phases are distinguished in terms of a many-body topological invariant and a quantized, experimentally accessible fractional corner charge that is robust against arbitrary, symmetry preserving edge manipulations. We support our claims by numerically studying the full counting statistics of the corner charge, finding a sharp distribution peaked around the quantized values. Our results allow for a direct comparison with experiments and represent a confirmation of theoretically predicted higher order topology in a strongly interacting system. Experimentally, the fractional corner charge can be observed in ultracold atomic settings using state of the art quantum gas microscopy.
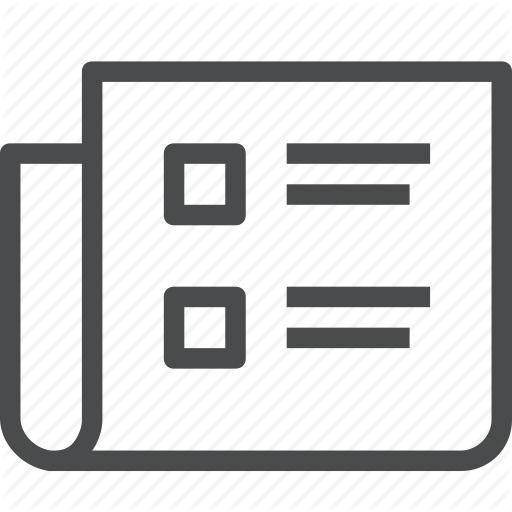
Parton theory of angle-resolved photoemission spectroscopy spectra in antiferromagnetic Mott insulators
A. Bohrdt, E. Demler, F. Pollmann, M. Knap, F. Grusdt
Physical Review B 102 (3), 035139 (2020).
Angle-resolved photoemission spectroscopy (ARPES) has revealed peculiar properties of mobile dopants in correlated antiferromagnets (AFMs). But, describing them theoretically, even in simplified toy models, remains a challenge. Here, we study ARPES spectra of a single mobile hole in the t-J model. Recent progress in the microscopic description of mobile dopants allows us to use a geometric decoupling of spin and charge fluctuations at strong couplings, from which we conjecture a one-to-one relation of the one-dopant spectral function and the spectrum of a constituting spinon in the undoped parent AFM. We thoroughly test this hypothesis for a single hole doped into a two-dimensional Heisenberg AFM by comparing our semianalytical predictions to previous quantum Monte Carlo results and our large-scale time-dependent matrix product state calculations of the spectral function. Our conclusion is supported by a microscopic trial wave function describing spinon-chargon bound states, which captures the momentum and t/J dependence of the quasiparticle residue. From our conjecture we speculate that ARPES measurements in the pseudogap phase of cuprates may directly reveal the Dirac-fermion nature of the constituting spinons. Specifically, we demonstrate that our trial wave function provides a microscopic explanation for the sudden drop of spectral weight around the nodal point associated with the formation of Fermi arcs, assuming that additional frustration suppresses long-range AFM ordering. We benchmark our results by studying the crossover from two to one dimension, where spinons and chargons are confined and deconfined, respectively.
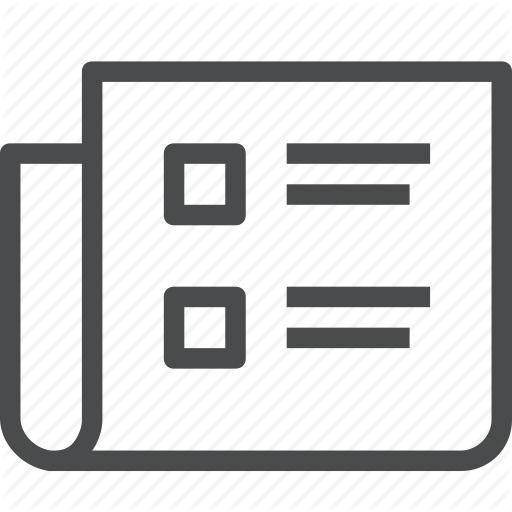
Many-body topological invariants from randomized measurements in synthetic quantum matter
A. Elben, J. Yu; G. Zhu, M. Hafezi, F. Pollmann, P. Zoller, B. Vermesch
Science Advances 6, eaaz3666 (2020).
Many-body topological invariants, as quantized highly nonlocal correlators of the many-body wave function, are at the heart of the theoretical description of many-body topological quantum phases, including symmetry-protected and symmetry-enriched topological phases. Here, we propose and analyze a universal toolbox of measurement protocols to reveal many-body topological invariants of phases with global symmetries, which can be implemented in state-of-the-art experiments with synthetic quantum systems, such as Rydberg atoms, trapped ions, and superconducting circuits. The protocol is based on extracting the many-body topological invariants from statistical correlations of randomized measurements, implemented with local random unitary operations followed by site-resolved projective measurements. We illustrate the technique and its application in the context of the complete classification of bosonic symmetry-protected topological phases in one dimension, considering in particular the extended Su-Schrieffer-Heeger spin model, as realized with Rydberg tweezer arrays.
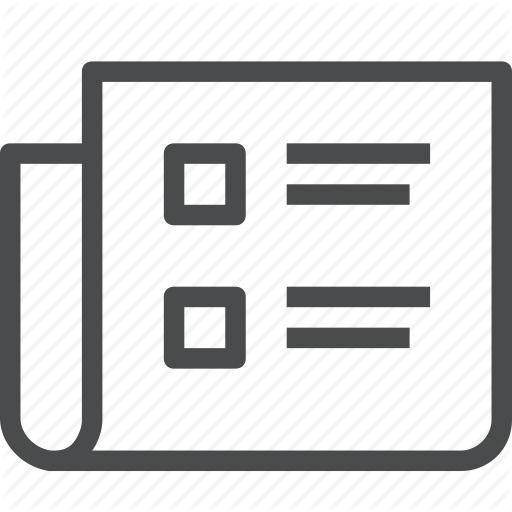
Statistical localization: From strong fragmentation to strong edge modes
T. Rakovszky, P. Sala, R. Verresen, M. Knap, F. Pollmann
Physical Review B 101 (12), 125126 (2020).
Certain disorder-free Hamiltonians can be nonergodic due to a strong fragmentation of the Hilbert space into disconnected sectors. Here, we characterize such systems by introducing the notion of "statistically localized integrals of motion" (SLIOM), whose eigenvalues label the connected components of the Hilbert space. SLIOMs are not spatially localized in the operator sense, but appear localized to subextensive regions when their expectation value is taken in typical states with a finite density of particles. We illustrate this general concept on several Hamiltonians, both with and without dipole conservation. Furthermore, we demonstrate that there exist perturbations which destroy these integrals of motion in the bulk of the system while keeping them on the boundary. This results in statistically localized strong zero modes, leading to infinitely long-lived edge magnetizations along with a thermalizing bulk, constituting the first example of such strong edge modes in a nonintegrable model. We also show that in a particular example, these edge modes lead to the appearance of topological string order in a certain subset of highly excited eigenstates. Some of our suggested models can be realized in Rydberg quantum simulators.
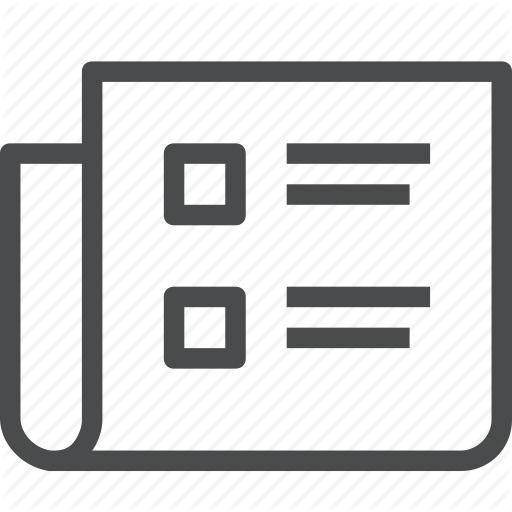
Ergodicity Breaking Arising from Hilbert Space Fragmentation in Dipole-Conserving Hamiltonians
P. Sala, T. Rakovszky, R. Verresen, M. Knap, F. Pollmann
Physical Review X 10 (1), 011047 (2020).
We show that the combination of charge and dipole conservation-characteristic of fracton systems-leads to an extensive fragmentation of the Hilbert space, which, in turn, can lead to a breakdown of thermalization. As a concrete example, we investigate the out-of-equilibrium dynamics of one-dimensional spin-1 models that conserve charge (total S-z) and its associated dipole moment. First, we consider a minimal model including only three-site terms and find that the infinite temperature autocorrelation saturates to a finite value-showcasing nonthermal behavior. The absence of thermalization is identified as a consequence of the strong fragmentation of the Hilbert space into exponentially many invariant subspaces in the local S-z basis, arising from the interplay of dipole conservation and local interactions. Second, we extend the model by including four-site terms and find that this perturbation leads to a weak fragmentation: The system still has exponentially many invariant subspaces, but they are no longer sufficient to avoid thermalization for typical initial states. More generally, for any finite range of interactions, the system still exhibits nonthermal eigenstates appearing throughout the entire spectrum. We compare our results to charge and dipole moment-conserving random unitary circuit models for which we reach identical conclusions.
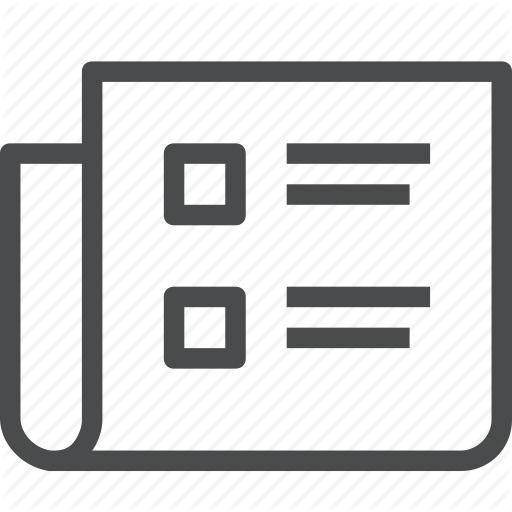
Quantum phases of a one-dimensional Majorana-Bose-Hubbard model
A. Roy, J. Hauschild, F. Pollmann
Physical Review B 101, 75419 (2020).
Majorana zero modes (MZM-s) occurring at the edges of a one-dimensional (1D), p-wave, spinless superconductor, in the absence of fluctuations of the phase of the superconducting order parameter, are quintessential examples of topologically protected zero-energy modes occurring at the edges of 1D symmetry-protected topological phases. In this work, we numerically investigate the fate of the topological phase in the presence of phase fluctuations using the density matrix renormalization group (DMRG) technique. To that end, we consider a one-dimensional array of MZM-s on mesoscopic superconducting islands at zero temperature. Cooper-pair and MZM-assisted single-electron tunneling, together with finite charging energy of the mesoscopic islands, give rise to a rich phase diagram of this model. We show that the system can be in either a Mott-insulating phase, a Luttinger liquid (LL) phase of Cooper pairs, or a second gapless phase. In contrast to the LL of Cooper pairs, this second phase is characterized by nonlocal string correlation functions which decay algebraically due to gapless charge-e excitations. The three phases are separated from each other by phase transitions of either Kosterlitz-Thouless or Ising type. Using a Jordan-Wigner transformation, we map the system to a generalized Bose-Hubbard model with two types of hopping and use DMRG to analyze the different phases and the phase transitions.
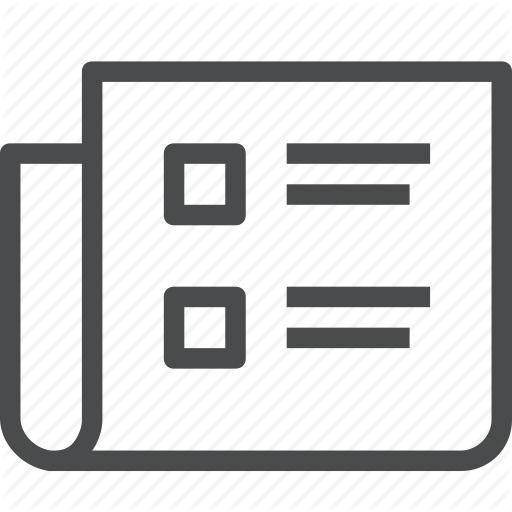
Isometric tensor network representation of string-net liquids
T. Soejima, K. Siva, N. Bultinck, S. Chatterjee, F. Pollmann, M.P. Zaletel
Physical Review B 101 (8), 085117 (2020).
Recently, a class of tensor networks called isometric tensor network states (isoTNS) was proposed which generalizes the canonical form of matrix product states to tensor networks in higher dimensions. While this ansatz allows for efficient numerical computations, it remained unclear which phases admit an isoTNS representation. In this work, we show that two-dimensional string-net liquids, which represent a wide variety of topological phases including discrete gauge theories, admit an exact isoTNS representation. We further show that the isometric form can be preserved after applying a finite-depth local quantum circuit. Taken together, these results show that long-range entanglement by itself is not an obstruction to isoTNS representation and suggest that all two-dimensional gapped phases with gappable edges admit an isoTNS representation.
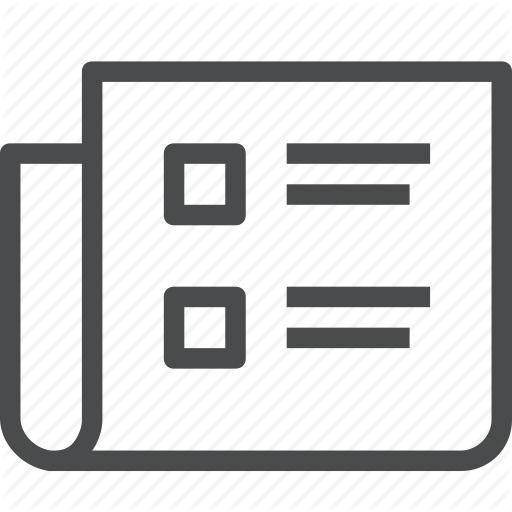
Isometric Tensor Network States in Two Dimensions
M.P. Zaletel, F. Pollmann
Physical Review Letters 124, 37201 (2020).
Tensor-network states (TNS) are a promising but numerically challenging tool for simulating two-dimensional (2D) quantum many-body problems. We introduce an isometric restriction of the TNS ansatz that allows for highly efficient contraction of the network. We consider two concrete applications using this ansatz. First, we show that a matrix-product state representation of a 2D quantum state can be iteratively transformed into an isometric 2D TNS. Second, we introduce a 2D version of the time-evolving block decimation algorithm for approximating of the ground state of a Hamiltonian as an isometric TNS—which we demonstrate for the 2D transverse field Ising model.
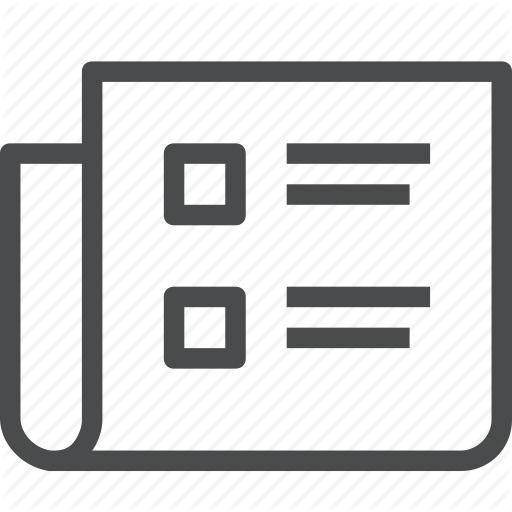
Dynamics of strongly interacting systems: From Fock-space fragmentation to many-body localization
G. De Tomasi, D. Hetterich, P. Sala, F. Pollman
Physical Review B 100 (21), 214313 (2019).
We study the t-V disordered spinless fermionic chain in the strong-coupling regime, t/V -> 0. Strong interactions highly hinder the dynamics of the model, fragmenting its Hilbert space into exponentially many blocks in system size. Macroscopically, these blocks can be characterized by the number of new degrees of freedom, which we refer to as movers. We focus on two limiting cases: blocks with only one mover and ones with a finite density of movers. The former many-particle block can be exactly mapped to a single-particle Anderson model with correlated disorder in one dimension. As a result, these eigenstates are always localized for any finite amount of disorder. The blocks with a finite density of movers, on the other side, show a many-body localized (MBL) transition that is tuned by the disorder strength. Moreover, we provide numerical evidence that its ergodic phase is diffusive at weak disorder. Approaching the MBL transition, we observe subdiffusive dynamics at finite timescales and find indications that this might be only a transient behavior before crossing over to diffusion.
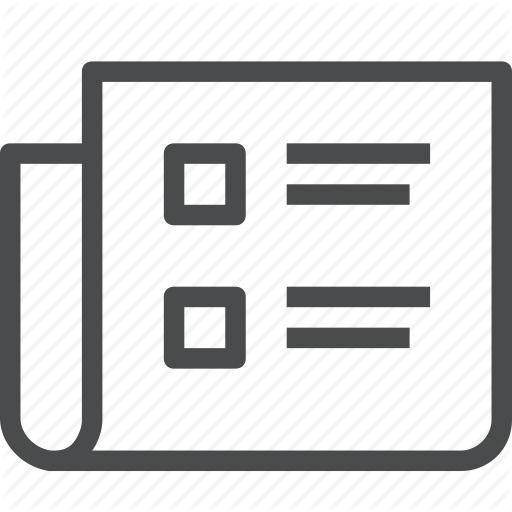
Entanglement growth after inhomogenous quenches
T. Rakovszky, C.W. von Keyserlingk, F. Pollmann
Physical Review B 100 (12), 125139 (2019).
We study the growth of entanglement in quantum systems with a conserved quantity exhibiting diffusive transport, focusing on how initial inhomogeneities are imprinted on the entropy. We propose a simple effective model, which generalizes the minimal cut picture of Jonay, Huse, and Nahum [arXiv:803.00089] in such a way that the line tension" of the cut depends on the local entropy density. In the case of noisy dynamics, this is described by the Kardar-Parisi-Zhang (KPZ) equation coupled to a diffusing field. We investigate the resulting dynamics and find that initial inhomogeneities of the conserved charge give rise to features in the entanglement profile, whose width and height both grow in time as alpha root t. In particular, for a domain wall quench, diffusion restricts entanglement growth to be S-VN less than or similar to root t. We find that for charge density wave initial states, these features in the entanglement profile are present even after the charge density has equilibrated. Our conclusions are supported by numerical results on random circuits and deterministic spin chains.
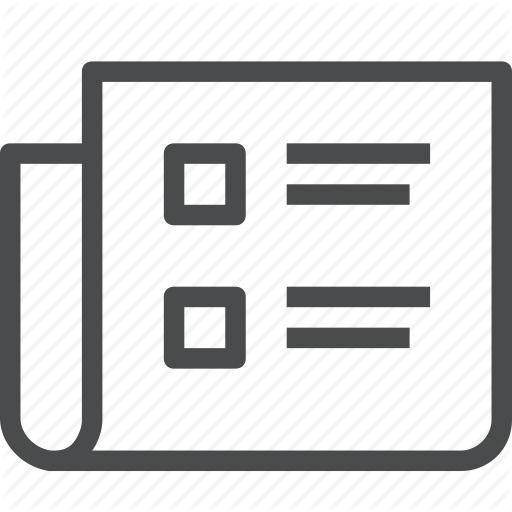
Matrix product states approaches to operator spreading in ergodic quantum systems
K. Hemery, F. Pollmann, D.J. Luitz
Physical Review B 100 (10), 104303 (2019).
We review different matrix-product-state (MPS) approaches to study the spreading of operators in generic nonintegrable quantum systems. As a common ground to all methods, we quantify this spreading by means of the Frobenius norm of the commutator of a spreading operator with a local operator, which is usually referred to as the out-of-time-order correlation (OTOC) function. We compare two approaches based on matrix-product states in the Schrodinger picture: the time-dependent block decimation (TEBD) and the time-dependent variational principle (TDVP), as well as TEBD based on matrix-product operators directly in the Heisenberg picture. The results of all methods are compared to numerically exact results using Krylov space exact time evolution. We find that for the Schrodinger picture, the TDVP algorithm performs better than the TEBD algorithm.
Moreover, the tails of the OTOC are accurately obtained both by TDVP MPS and TEBD MPO. They are in very good agreement with exact results at short times, and appear to be converged in bond dimensions even at longer times. However, the growth and saturation regimes are not well captured by either of the methods.
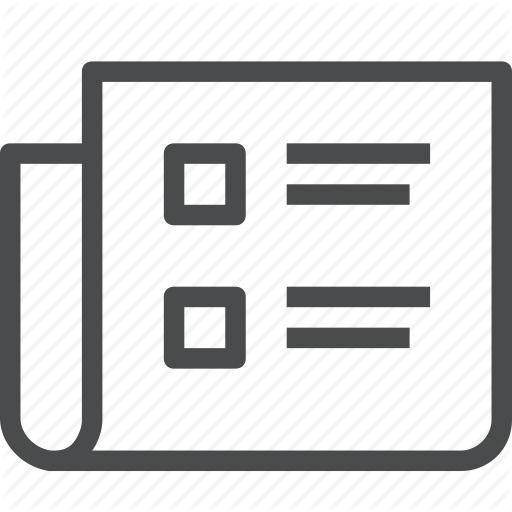
Signatures of information scrambling in the dynamics of the entanglement spectrum
T. Rakovszky, S. Gopalakrishnan, S.A. Parameswaran, F. Pollmann
Physical Review B 100 (12), 125115 (2019).
We examine the time evolution of the entanglement spectrum of a small subsystem of a nonintegrable spin chain following a quench from a product state. We identify signatures in this entanglement spectrum of the distinct dynamical velocities (related to entanglement and operator spreading) that control thermalization. We show that the onset of level repulsion in the entanglement spectrum occurs on different timescales depending on the "entanglement energy," and that this dependence reflects the shape of the operator front. Level repulsion spreads across the entire entanglement spectrum on a timescale that is parametrically shorter than that for full thermalization of the subsystem. This timescale is also close to when the mutual information between individual spins at the ends of the subsystem reaches its maximum. We provide an analytical understanding of this phenomenon and show supporting numerical data for both random unitary circuits and a microscopic Hamiltonian.
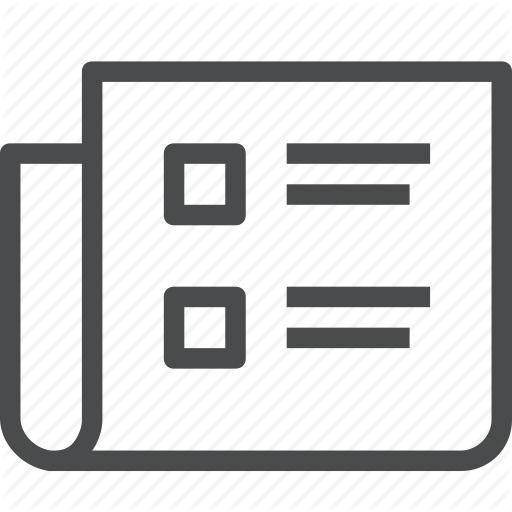
Polarization plateaus in hexagonal water ice Ih
M. Gohlke, R. Moessner, F. Pollmann
Physical Review B 100, 14206 (2019).
The protons in water ice are subject to so-called ice rules resulting in an extensive ground-state degeneracy. We study how an external electric field reduces this ground-state degeneracy in hexagonal water ice Ih within a minimal model. We observe polarization plateaus when the field is aligned along the [001] and [010] directions. In each case, one plateau occurs at intermediate polarization with reduced but still extensive degeneracy. The remaining ground states can be mapped to dimer models on the honeycomb and the square lattice, respectively. Upon tilting the external field, we observe an order-disorder transition of Kasteleyn type into a plateau at saturated polarization and vanishing entropy. This transition is investigated analytically using the Kasteleyn matrix and numerically using a modified directed-loop Monte Carlo simulation. The protons in both cases exhibit algebraically decaying correlations. Moreover, the features of the static structure factor are discussed.
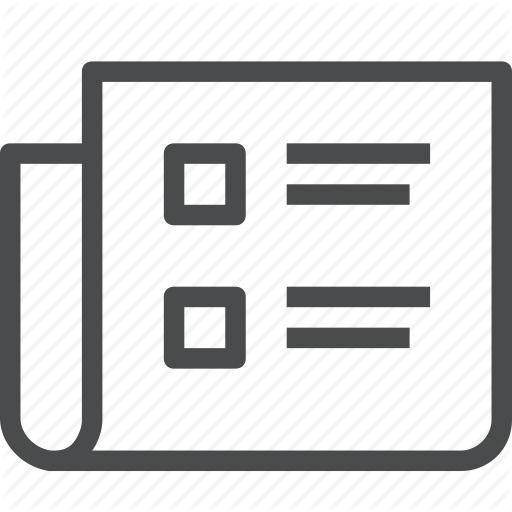
Emergent Glassy Dynamics in a Quantum Dimer Model
J. Feldmeier, F. Pollmann, and M. Knap.
Physical Review Letters 123, 040601 (2019).
We consider the quench dynamics of a two-dimensional quantum dimer model and determine the role of its kinematic constraints. We interpret the nonequilibrium dynamics in terms of the underlying equilibrium phase transitions consisting of a Berezinskii-Kosterlitz-Thouless (BKT) transition between a columnar ordered valence bond solid (VBS) and a valence bond liquid (VBL), as well as a first-order transition between a staggered VBS and the VBL. We find that quenches from a columnar VBS are ergodic and both order parameters and spatial correlations quickly relax to their thermal equilibrium. By contrast, the staggered side of the first-order transition does not display thermalization on numerically accessible timescales. Based on the model’s kinematic constraints, we uncover a mechanism of relaxation that rests on emergent, highly detuned multidefect processes in a staggered background, which gives rise to slow, glassy dynamics at low temperatures even in the thermodynamic limit.
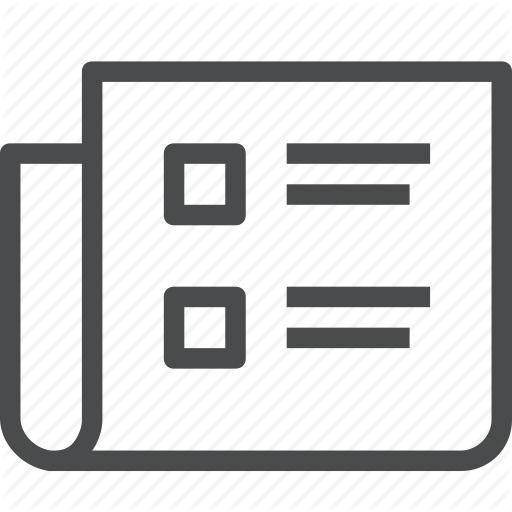
Efficiently solving the dynamics of many-body localized systems at strong disorder
G. De Tomasi, F. Pollmann, M. Heyl
Physical Review B 99, 241114 (R) (2019).
We introduce a method to efficiently study the dynamical properties of many-body localized systems in the regime of strong disorder and weak interactions. Our method reproduces qualitatively and quantitatively the time evolution with a polynomial effort in system size and independent of the desired time scales. We use our method to study quantum information propagation, correlation functions, and temporal fluctuations in one- and two-dimensional many-body localization systems. Moreover, we outline strategies for a further systematic improvement of the accuracy and we point out relations of our method to recent attempts to simulate the time dynamics of quantum many-body systems in classical or artificial neural networks.
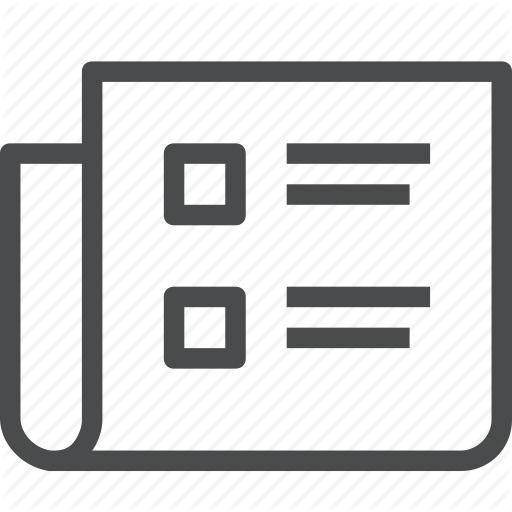
Sub-ballistic Growth of Renyi Entropies due to Diffusion
T. Rakovszky, F. Pollmann, C.W. von Keyserlingk
Physical Review Letters 122 (25), 250602 (2019).
We investigate the dynamics of quantum entanglement after a global quench and uncover a qualitative difference between the behavior of the von Neumann entropy and higher Renyi entropies. We argue that the latter generically grow sub-ballistically, as proportional to root t, in systems with diffusive transport. We provide strong evidence for this in both a U(1) symmetric random circuit model and in a paradigmatic nonintegrable spin chain, where energy is the sole conserved quantity. We interpret our results as a consequence of local quantum fluctuations in conserved densities, whose behavior is controlled by diffusion, and use the random circuit model to derive an effective description. We also discuss the late-time behavior of the second Renyi entropy and show that it exhibits hydrodynamic tails with three distinct power laws occurring for different classes of initial states.
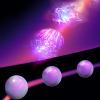
Avoided quasiparticle decay from strong quantum interactions
R. Verresen, R. Moessner, F. Pollmann.
Nature Physics 15, 750-753 (2019).
Quantum states of matter—such as solids, magnets and topological phases—typically exhibit collective excitations (for example, phonons, magnons and anyons). These involve the motion of many particles in the system, yet, remarkably, act like a single emergent entity—a quasiparticle. Known to be long lived at the lowest energies, quasiparticles are expected to become unstable when encountering the inevitable continuum of many-particle excited states at high energies, where decay is kinematically allowed. Although this is correct for weak interactions, we show that strong interactions generically stabilize quasiparticles by pushing them out of the continuum. This general mechanism is straightforwardly illustrated in an exactly solvable model. Using state-of-the-art numerics, we find it at work in the spin-1/2 triangular-lattice Heisenberg antiferromagnet (TLHAF). This is surprising given the expectation of magnon decay in this paradigmatic frustrated magnet. Turning to existing experimental data, we identify the detailed phenomenology of avoided decay in the TLHAF material Ba3CoSb2O9, and even in liquid helium, one of the earliest instances of quasiparticle decay. Our work unifies various phenomena above the universal low-energy regime in a comprehensive description. This broadens our window of understanding of many-body excitations, and provides a new perspective for controlling and stabilizing quantum matter in the strongly interacting regime.