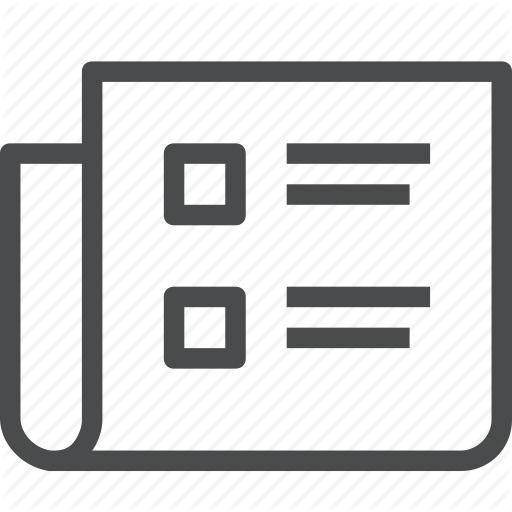
Spatially Tunable Spin Interactions in Neutral Atom Arrays
L. M. Steinert, P. Osterholz, R. Eberhard, L. Festa, N. Lorenz, Z. J. Chen, A. Trautmann, C. Gross
Physical Review Letters 130 (24), 243001 (2023).
Analog quantum simulations with Rydberg atoms in optical tweezers routinely address strongly correlated many-body problems due to the hardware-efficient implementation of the Hamiltonian. Yet, their generality is limited, and flexible Hamiltonian-design techniques are needed to widen the scope of these simulators. Here we report on the realization of spatially tunable interactions for XYZ models implemented by two-color near-resonant coupling to Rydberg pair states. Our results demonstrate the unique opportunities of Rydberg dressing for Hamiltonian design in analog quantum simulators.
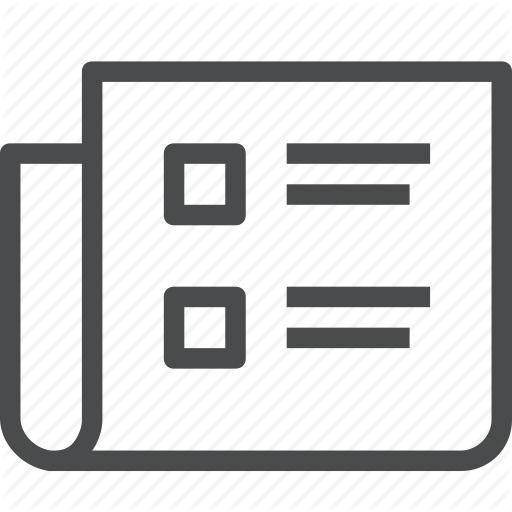
Realizing the symmetry-protected Haldane phase in Fermi-Hubbard ladders
P. Sompet, S. Hirthe, D. Bourgund, T. Chalopin, J. Bibo, J. Koepsell, P. Bojovic, R. Verresen, F. Pollmann, G. Salomon, C. Gross, T. A. Hilker, I. Bloch
Nature 606 (7914), 484-+ (2022).
Topology in quantum many-body systems has profoundly changed our understanding of quantum phases of matter. The model that has played an instrumental role in elucidating these effects is the antiferromagnetic spin-1 Haldane chain(1,2). Its ground state is a disordered state, with symmetry-protected fourfold-degenerate edge states due to fractional spin excitations. In the bulk, it is characterized by vanishing two-point spin correlations, gapped excitations and a characteristic non-local order parameter(3,4). More recently it has been understood that the Haldane chain forms a specific example of a more general classification scheme of symmetry-protected topological phases of matter, which is based on ideas connected to quantum information and entanglement(5-7). Here, we realize a finite-temperature version of such a topological Haldane phase with Fermi-Hubbard ladders in an ultracold-atom quantum simulator. We directly reveal both edge and bulk properties of the system through the use of single-site and particle-resolved measurements, as well as non-local correlation functions. Continuously changing the Hubbard interaction strength of the system enables us to investigate the robustness of the phase to charge (density) fluctuations far from the regime of the Heisenberg model, using a novel correlator.
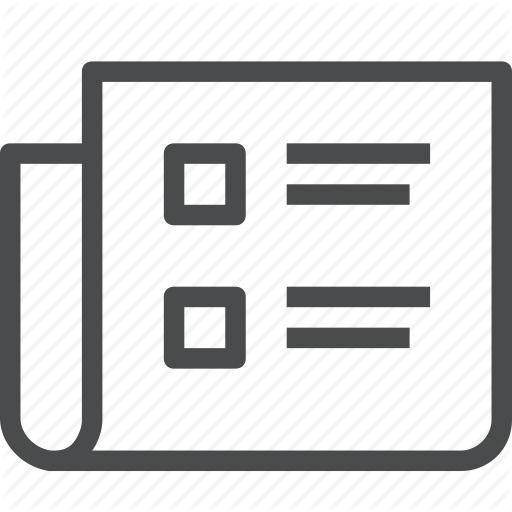
Realizing Distance-Selective Interactions in a Rydberg-Dressed Atom Array
S. Hollerith, K. Srakaew, D. Wei, A. Rubio-Abadal, D. Adler, P. Weckesser, A. Kruckenhauser, V. Walther, R. van Bijnen, J. Rui, C. Gross, I. Bloch, J. Zeiher
Physical Review Letters 128 (11), 113602 (2022).
Measurement-based quantum computing relies on the rapid creation of large-scale entanglement in a register of stable qubits. Atomic arrays are well suited to store quantum information, and entanglement can be created using highly-excited Rydberg states. Typically, isolating pairs during gate operation is difficult because Rydberg interactions feature long tails at large distances. Here, we engineer distance-selective interactions that are strongly peaked in distance through off-resonant laser coupling of molecular potentials between Rydberg atom pairs. Employing quantum gas microscopy, we verify the dressed interactions by observing correlated phase evolution using many-body Ramsey interferometry. We identify atom loss and coupling to continuum modes as a limitation of our present scheme and outline paths to mitigate these effects, paving the way towards the creation of large-scale entanglement.
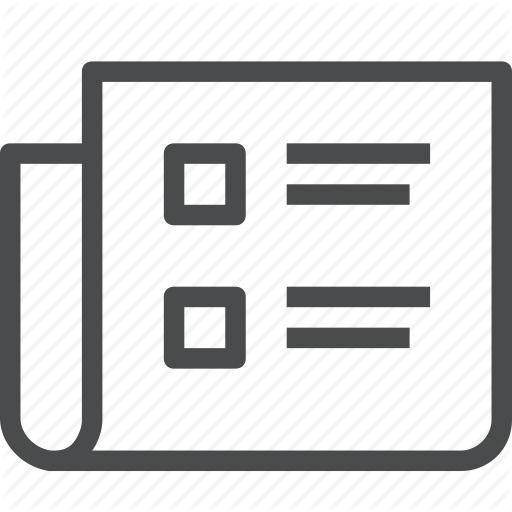
Blackbody-radiation-induced facilitated excitation of Rydberg atoms in optical tweezers
L. Festa, N. Lorenz, L. M. Steinert, Z. J. Chen, P. Osterholz, R. Eberhard, C. Gross
Physical Review A 105 (1), 13109 (2022).
Blackbody radiation, omnipresent at room temperature, couples nearby Rydberg states. The resulting state mixture features strong dipolar interactions, which may induce dephasing in a Rydberg many-body system. Here we report on a single atom resolved study of this state contamination and the emerging pairwise interactions in optical tweezers. For near-resonant laser detuning we observe characteristic correlations with a length scale set by the dipolar interaction. Our study reveals the microscopic origin of avalanche excitation observed in previous experiments.
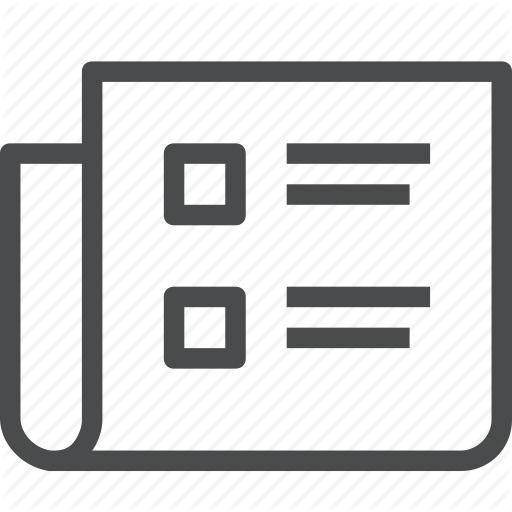
Quantum gas microscopy for single atom and spin detection
C. Gross, W. S. Bakr
Nature Physics 17 (12), 1316-1323 (2021).
Ultracold gases provide a platform for idealized realizations of many-body systems. Thanks to recent advances in quantum gas microscopy, collective quantum phenomena can be probed with single-site resolution. A particular strength of ultracold quantum gases is the range of versatile detection methods that are available. As they are based on atom-light interactions, the whole quantum optics toolbox can be used to tailor the detection process to the specific scientific question to be explored in the experiment. Common methods include time-of-flight measurements to access the momentum distribution of the gas, the use of cavities to monitor global properties of the quantum gas with minimal disturbance, and phase-contrast or high-intensity absorption imaging to obtain local real-space information in high-density settings. Even the ultimate limit of detecting each and every atom locally has been realized in two dimensions using so-called quantum gas microscopes. In fact, these microscopes have not only revolutionized detection-they have also revolutionized the control of lattice gases. Here, we provide a short overview of quantum gas microscopy, highlighting the new observables it can access as well as key experiments that have been enabled by its development.
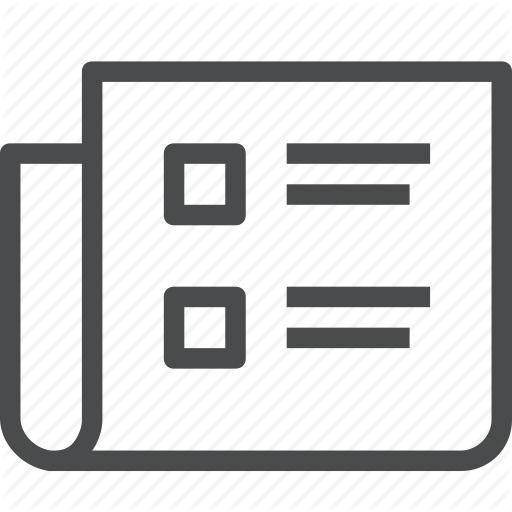
Microscopic evolution of doped Mott insulators from polaronic metal to Fermi liquid
J. Koepsell, D. Bourgund, P. Sompet, S. Hirthe, A. Bohrdt, Y. Wang, F. Grusdt, E. Demler, G. Salomon, C. Gross, I. Bloch
Science 374 (6563), 82-+ (2021).
The competition between antiferromagnetism and hole motion in two-dimensional Mott insulators lies at the heart of a doping-dependent transition from an anomalous metal to a conventional Fermi liquid. We observe such a crossover in Fermi-Hubbard systems on a cold-atom quantum simulator and reveal the transformation of multipoint correlations between spins and holes upon increasing doping at temperatures around the superexchange energy. Conventional observables, such as spin susceptibility, are furthermore computed from the microscopic snapshots of the system. Starting from a magnetic polaron regime, we find the system evolves into a Fermi liquid featuring incommensurate magnetic fluctuations and fundamentally altered correlations. The crossover is completed for hole dopings around 30%. Our work benchmarks theoretical approaches and discusses possible connections to lowertemperature phenomena.
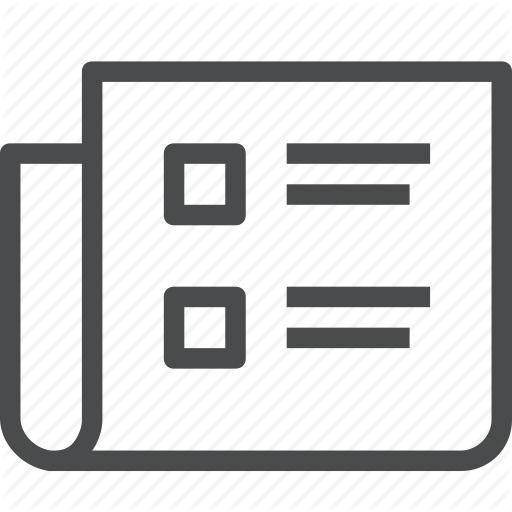
Microscopic electronic structure tomography of Rydberg macrodimers
S. Hollerith, J. Rui, A. Rubio-Abadal, K. Srakaew, D. Wei, J. Zeiher, C. Gross, I. Bloch
Physical Review Research 3 (1), 13252 (2021).
Precise control and study of molecules is challenging due to the variety of internal degrees of freedom and local coordinates that are typically not controlled in an experiment. Employing quantum gas microscopy to position and resolve the atoms in Rydberg macrodimer states solves most of these challenges and enables unique access to the molecular frame. Here, we demonstrate this approach and present photoassociation studies in which the molecular orientation relative to an applied magnetic field, the polarization of the excitation light, and the initial atomic state are fully controlled. The observed dependencies allow for an electronic structure tomography of the molecular state. We additionally observe an orientation-dependent Zeeman shift, and we reveal a significant influence on it caused by the hyperfine interaction of the macrodimer state. Finally, we demonstrate control over the electrostatic binding potential by engineering a gap between two crossing pair potentials. Our results establish macrodimers as the most sensitive tool to benchmark Rydberg interaction potentials, and they open new perspectives for improving Rydberg dressing schemes.
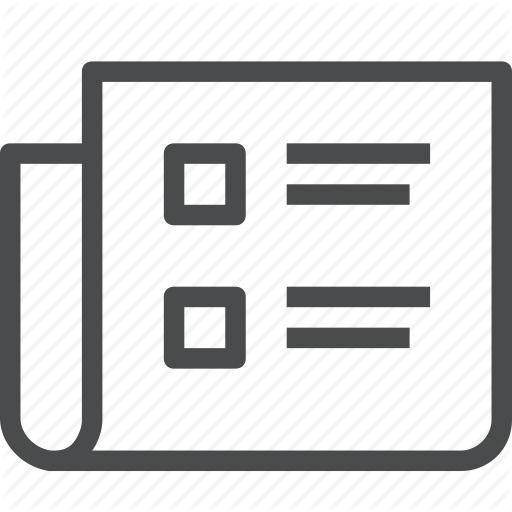
Raman sideband cooling in optical tweezer arrays for Rydberg dressing
N. Lorenz, L. Festa, L. M. Steinert, C. Gross
Scipost Physics 10 (3), 52 (2021).
Single neutral atoms trapped in optical tweezers and laser-coupled to Rydberg states provide a fast and flexible platform to generate configurable atomic arrays for quantum simulation. The platform is especially suited to study quantum spin systems in various geometries. However, for experiments requiring continuous trapping, inhomogeneous light shifts induced by the trapping potential and temperature broadening impose severe limitations. Here we show how Raman sideband cooling allows one to overcome those limitations, thus, preparing the stage for Rydberg dressing in tweezer arrays.
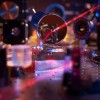
A subradiant optical mirror formed by a single structured atomic layer
J. Rui, D. V. Wei, A. Rubio-Abadal, S. Hollerith, J. Zeiher, D. M. Stamper-Kurn, C. Gross, I. Bloch
Nature 583 (7816), 369-+ (2020).
Versatile interfaces with strong and tunable light-matter interactions are essential for quantum science(1)because they enable mapping of quantum properties between light and matter(1). Recent studies(2-10)have proposed a method of controlling light-matter interactions using the rich interplay of photon-mediated dipole-dipole interactions in structured subwavelength arrays of quantum emitters. However, a key aspect of this approach-the cooperative enhancement of the light-matter coupling strength and the directional mirror reflection of the incoming light using an array of quantum emitters-has not yet been experimentally demonstrated. Here we report the direct observation of the cooperative subradiant response of a two-dimensional square array of atoms in an optical lattice. We observe a spectral narrowing of the collective atomic response well below the quantum-limited decay of individual atoms into free space. Through spatially resolved spectroscopic measurements, we show that the array acts as an efficient mirror formed by a single monolayer of a few hundred atoms. By tuning the atom density in the array and changing the ordering of the particles, we are able to control the cooperative response of the array and elucidate the effect of the interplay of spatial order and dipolar interactions on the collective properties of the ensemble. Bloch oscillations of the atoms outside the array enable us to dynamically control the reflectivity of the atomic mirror. Our work demonstrates efficient optical metamaterial engineering based on structured ensembles of atoms(4,8,9)and paves the way towards controlling many-body physics with light(5,6,11)and light-matter interfaces at the single-quantum level(7,10). A single two-dimensional array of atoms trapped in an optical lattice shows a tunable cooperative subradiant optical response, acting as a single-monolayer optical mirror with controllable reflectivity.
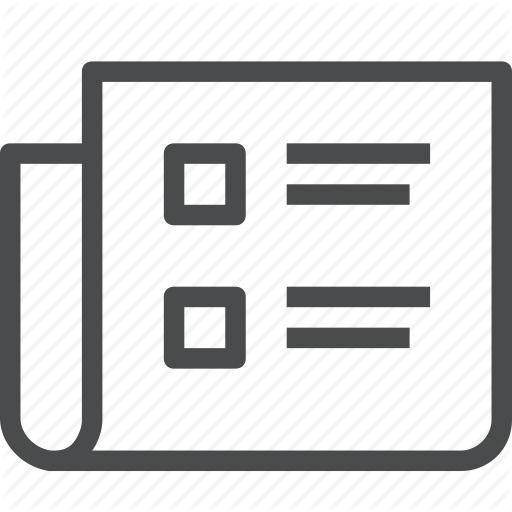
Robust Bilayer Charge Pumping for Spin- and Density-Resolved Quantum Gas Microscopy
J. Koepsell, S. Hirthe, D. Bourgund, P. Sompet, J. Vijayan, G. Salomon, C. Gross, I. Bloch
Physical Review Letters 125 (1), 10403 (2020).
Quantum gas microscopy has emerged as a powerful new way to probe quantum many-body systems at the microscopic level. However, layered or efficient spin-resolved readout methods have remained scarce as they impose strong demands on the specific atomic species and constrain the simulated lattice geometry and size. Here we present a novel high-fidelity bilayer readout, which can be used for full spin- and density-resolved quantum gas microscopy of two-dimensional systems with arbitrary geometry. Our technique makes use of an initial Stern-Gerlach splitting into adjacent layers of a highly stable vertical superlattice and subsequent charge pumping to separate the layers by 21 mu m. This separation enables independent high-resolution images of each layer. We benchmark our method by spin- and density-resolving two-dimensional Fermi-Hubbard systems. Our technique furthermore enables the access to advanced entropy engineering schemes, spectroscopic methods, or the realization of tunable bilayer systems.
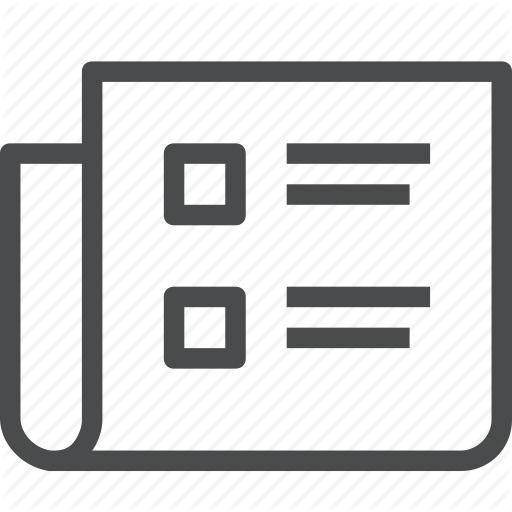
Floquet Prethermalization in a Bose-Hubbard System
A. Rubio-Abadal, M. Ippoliti, S. Hollerith, D. Wei, J. Rui, S. L. Sondhi, V. Khemani, C. Gross, I. Bloch
Physical Review X 10 (2), 21044 (2020).
"Periodic driving has emerged as a powerful tool in the quest to engineer new and exotic quantum phases. While driven many-body systems are generically expected to absorb energy indefinitely and reach an infinite-temperature state, the rate of heating can be exponentially suppressed when the drive frequency is large compared to the local energy scales of the system-leading to long-lived ""prethermal"" regimes. In this work, we experimentally study a bosonic cloud of ultracold atoms in a driven optical lattice and identify such a prethermal regime in the Bose-Hubbard model. By measuring the energy absorption of the cloud as the driving frequency is increased, we observe an exponential-in-frequency reduction of the heating rate persisting over more than 2 orders of magnitude. The tunability of the lattice potentials allows us to explore one- and two-dimensional systems in a range of different interacting regimes. Alongside the exponential decrease, the dependence of the heating rate on the frequency displays features characteristic of the phase diagram of the Bose-Hubbard model, whose understanding is additionally supported by numerical simulations in one dimension. Our results show experimental evidence of the phenomenon of Floquet prethermalization and provide insight into the characterization of heating for driven bosonic systems."
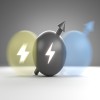
Time-resolved observation of spin-charge deconfinement in fermionic Hubbard chains
J. Vijayan, P. Sompet, G. Salomon, J. Koepsell, S. Hirthe, A. Bohrdt, F. Grusdt, I. Bloch, C. Gross
Science 367 (6474), 186-+ (2020).
Elementary particles carry several quantum numbers, such as charge and spin. However, in an ensemble of strongly interacting particles, the emerging degrees of freedom can fundamentally differ from those of the individual constituents. For example, one-dimensional systems are described by independent quasiparticles carrying either spin (spinon) or charge (holon). Here, we report on the dynamical deconfinement of spin and charge excitations in real space after the removal of a particle in Fermi-Hubbard chains of ultracold atoms. Using space- and time-resolved quantum gas microscopy, we tracked the evolution of the excitations through their signatures in spin and charge correlations. By evaluating multipoint correlators, we quantified the spatial separation of the excitations in the context of fractionalization into single spinons and holons at finite temperatures.